Pioneered by Stanford engineers a few years ago, resistive random-access memory (RRAM) is an emerging type of computer memory based on a new semiconductor material that relies on temperature and voltage to store data. While it's proven faster and more energy efficient than current technology, exactly how RRAM works remained a mystery. Now, a Stanford team has used a new tool to investigate the chips and found the optimal temperature range was lower than expected, paving the way for more efficient memory.
Your garden-variety computer chip operates on a two-dimensional plane, with CPU and memory communicating back and forth through the data bus. The problem is that data buses aren't advancing as fast as other components, so crunching large amounts of data can slow down the rest of the system.
RRAM, and the semiconductor that it's made of, can help overcome the issue by allowing chips to be stacked on top of each other, bringing the memory and logic components closer together in a way that silicon devices can't replicate. These 3D "high rise" chips could make for faster and more energy efficient solutions for tackling the challenges of Big Data and for extending the battery life of future mobile devices.
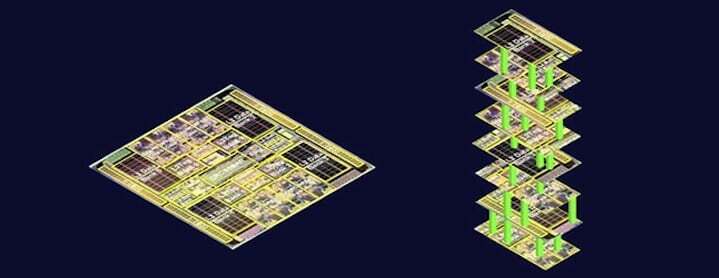
In order to make these chips a reality, the Stanford engineers needed to better understand how RRAM functions. They already knew the basics: in their natural state, RRAM materials are insulators, resisting the flow of electrons, but zapping them with an electric field can cause them to open a path (called a filament) for electrons, with another jolt switching them back. Alternating between those two states can form the basis of binary code: no signal (thanks to insulation) represents a zero, while electrons passing through represents a one.
These filaments are opened and closed as a result of the temperature of the materials, which is raised as electrons pass through it. Just how hot the materials needed to be to cause the switch was the unknown factor.
"We need much more precise information about the fundamental behavior of RRAM before we can hope to produce reliable devices," says Philip Wong, the Stanford electrical engineer who led the study.
Without a way to measure how much heat is generated by a jolt of electricity, the researchers aimed to remove voltage from the equation, instead heating RRAM chips using a hot plate-like device called a micro thermal stage. By monitoring when electrons started to flow through the filaments, the team was able to measure the temperatures needed for the materials to form filaments – and were pleasantly surprised at how low they turned out to be.
The chips were heated to various temperatures, from a comfortable 80° F (26.7° C) right up to a fiery 1,520° F (826.7° C), and the researchers found that the optimal range was, surprisingly, at the low end of the scale. The filaments were forming the most efficiently at temperatures between 80° F and 260º F (26.7 and 126.7° C), far lower than the previous estimate of 1,160° F (626.7° C). As a result, future RRAM devices would need less electricity to generate these temperatures, making them more energy efficient.
RRAM may not be ready for consumer devices any time soon, but the researchers say that this discovery will help speed development along, by improving our understanding of the technology and providing a more solid base for future work to build on.
"Now we can use voltage and temperature as design inputs in a predictive manner and that is going to enable us to design a better memory device," says Ziwen Wang, another member of the team.
The research will be presented at the IEEE International Electron Devices Meeting (IEDM) in San Francisco this week.
Source: Stanford University