These are some absolutely wild ideas, so before we get started, it's important to know that Michael Levin is no fringe scientist or crackpot. He's a director of the Allen Discovery Center at Tufts University, as well as the Tufts Center for Regenerative and Developmental Biology, and a co-director of the Institute for Computationally Designed Organisms. He's co-authored several hundred research papers, with nearly 30,000 citations, and has a list of distinguishing awards as long as your arm.
All of which is to say: we can see why Levin's work might have flown under a lot of folks' radars; this quick summary is gonna get pretty 'out there.' But over more than two decades, he's earned the right to be taken seriously.
It's also important to note that Levin is just one leading researcher in a broad and expanding field – he just provides an excellent pathway into these ideas thanks to a number of clear, well-considered presentations and interviews.
Cellular intelligence: Upending our understanding of DNA
So with that in mind, let's start out by upending one commonly held notion: that DNA is the blueprint from which our bodies are built; the set of instructions that tells stem cells where to grow and what to become, in order to assemble a biological organism. In an interview with "Head of TED" Chris Anderson in 2021, Levin explains one problem with that notion.
"A tadpole, as it becomes a frog, has to rearrange its face," says Levin. "The eyes, the nostrils, the jaws, everything has to move. One way to think about that used to be that you have a sort of hardwired set of movements, where all these things move around, and then you get your frog. But a few years ago, we found a pretty amazing phenomenon.
"If you make a so-called 'Picasso frog' – these are tadpoles where the jaws might be off to the side, the eyes are up here, the nostrils are moved, everything is shifted – these tadpoles make largely normal frog faces. That's amazing – all the organs start off in abnormal positions, yet they still end up making a pretty good frog face.
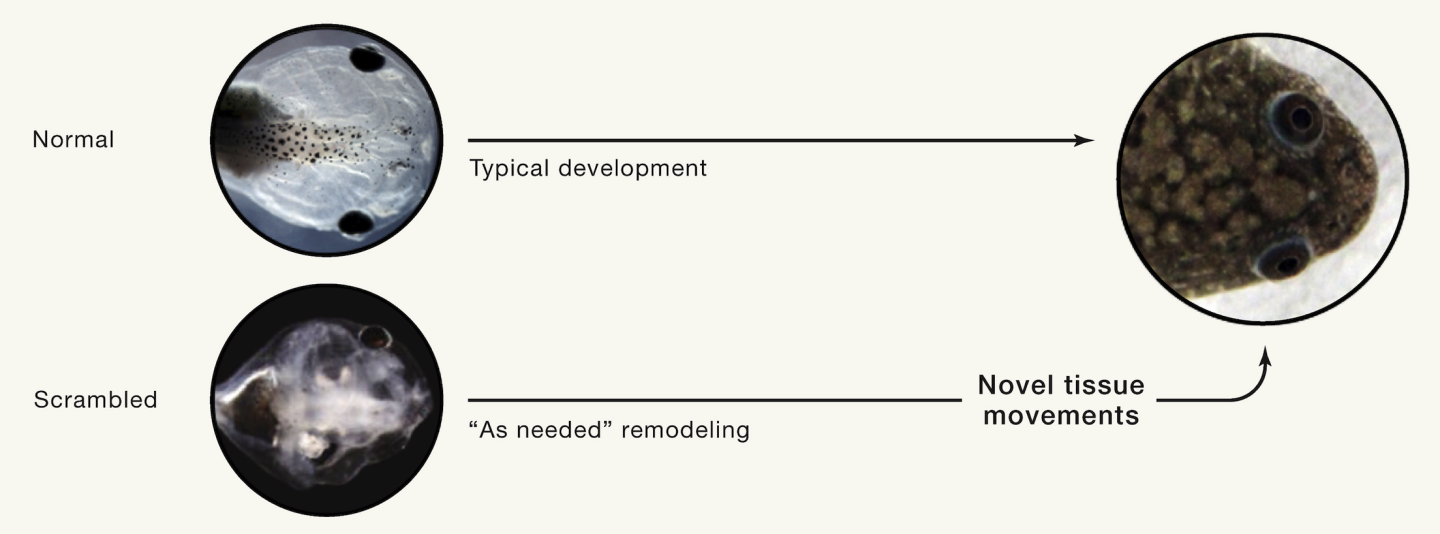
"So this system, like many living systems, is not a set of hardwired movements; it actually works to reduce the error between what's going on now, and what it knows is a correct frog face configuration. This kind of decision making, that involves flexible responses to new circumstances? In other contexts, we would call this intelligence."
So if it's not DNA communicating to these cells via biochemical signals, then how do these cells know where they are, and what to do next?
Bioelectricity: It's not just for nerve cells
"They certainly do communicate biochemically and via physical forces," says Levin. "But there's something else going on that's extremely interesting. It's basically called bioelectricity – non-neural bioelectricity. It turns out that all cells – not just nerves, but all cells in your body – communicate with each other using electrical signals."
Levin and his team began tracking these electrical signals in frog embryos as they developed, and saw that as soon as cells begin to divide in a new organism, they start to form electrical networks. And over many years, they developed a new understanding: the DNA, in some sense, is not the software routine that builds your body. It's more like the hardware that an intelligent system runs on.
"People are not enamored of computer analogies in biology, and in many ways they're bad," Levin tells Arizona State University Professor Andrew Maynard in another interview earlier this year. "But this one, I think, is a good one. What the genome encodes is the hardware – it tells every cell what kind of microscopic hardware it has to play with. Those are the proteins it has. Everything that happens after that is software.
"And that hardware is reprogrammable. The genome does not directly specify your shape. It doesn't shape the content of the memories of your body networks. What it gives you is some amazing hardware that does some stuff by default, out of the box, but it's also highly reprogrammable."
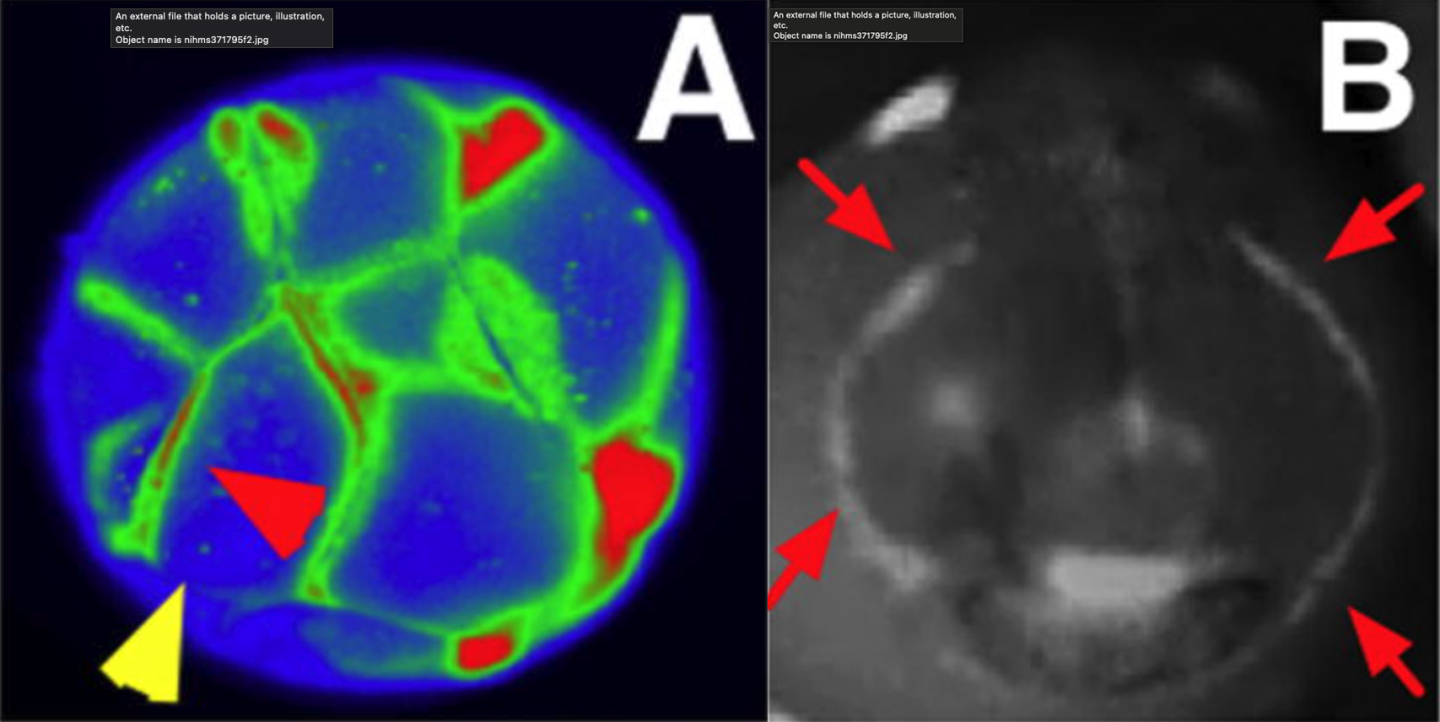
"The cells form electrical networks, and these networks process information, including pattern memories," he tells Anderson back in 2021. "They include representations of large-scale anatomical structures, where various organs will go, where the different axes of the animal – front and back, head and tail – will be. These are literally held in the electrical circuits across large tissues in the same way that brains hold other kinds of memories and learning."
The multi-headed flatworm experiments
Just like any other computing system, it seems these bioelectrical cell networks can be hacked.
"You can take a step back," Levin tells Andersen, "and give [a cellular network] stimuli or inputs, the way that you'd give them to a reprogrammable computer, and cause the cellular network to do something completely different than it would otherwise have done. These bioelectrical signals give us an entry point directly into the software that guides large scale anatomy – which is a very different approach to medicine than rewiring specific pathways inside every cell."
To demonstrate, Levin and his team chose the planarian – a freshwater-dwelling flatworm with the remarkable ability to fully regenerate body parts when they're cut off. These creatures have a complete brain, as well as numerous internal organs, but you can chop them into more or less unlimited pieces, and each piece will regenerate everything it's missing in order to form a complete worm.
"This is a system where every single piece knows what a complete planarian looks like," says Levin, "and it can rebuild the right organs in the right places, and then it stops."
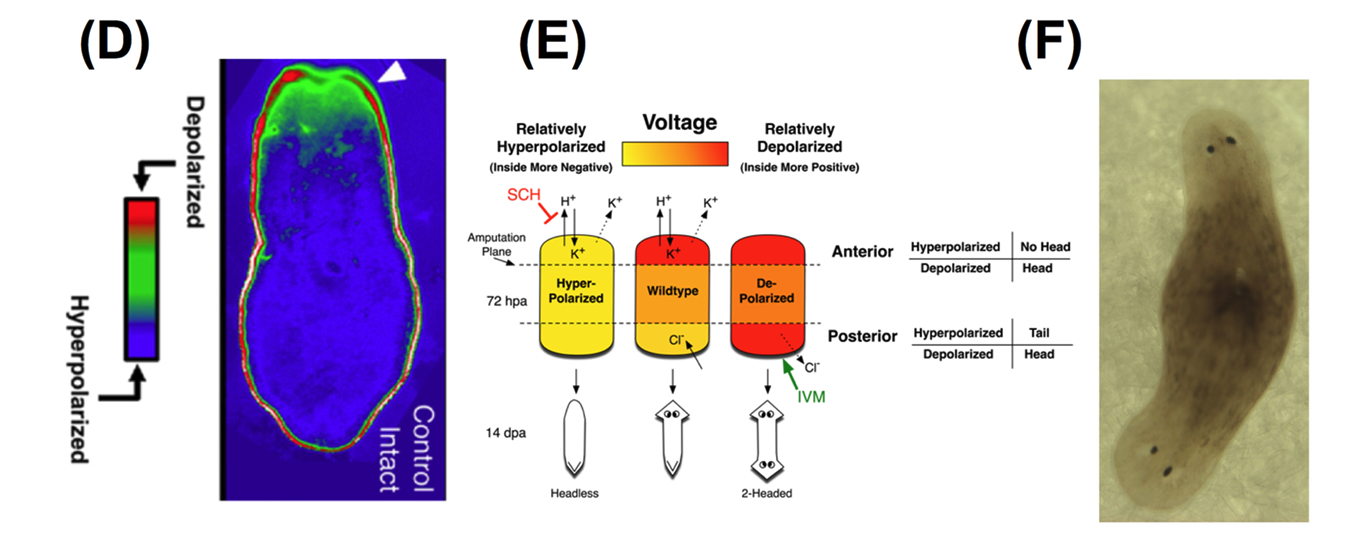
Levin and his team chopped off the head and tails of these flatworms, and measured an electrical gradient between the head and tail stumps, and ran some experiments to see if manipulating this electrical gradient would confuse the cellular networks into building the wrong parts.
"We don't apply electricity," he tells Andersen. "What we do is turn on and off the little transistors – they're actually ion channel proteins that every cell natively uses to set up its electrical state. And when you do this, you can shift that circuit to a state that says 'build two heads,' or in fact, 'build no heads.' And what you're seeing here are real worms that have either two, or no heads, because that electrical map is what the cells are using to decide what to do.
"And having generated these, we did a completely crazy experiment. You take one of these two-headed worms, and you chop off both heads, leaving just the normal middle fragment. Keep in mind, these animals have not been genetically edited... Their genomic sequence is completely wild type... And the standard paradigm would say, if you've gotten rid of this extra tissue, and the genome is edited, it should make a perfectly normal worm. And the amazing thing is, that's not what happens. These worms, when cut again and again, in plain water, they continue to regenerate as two-headed.
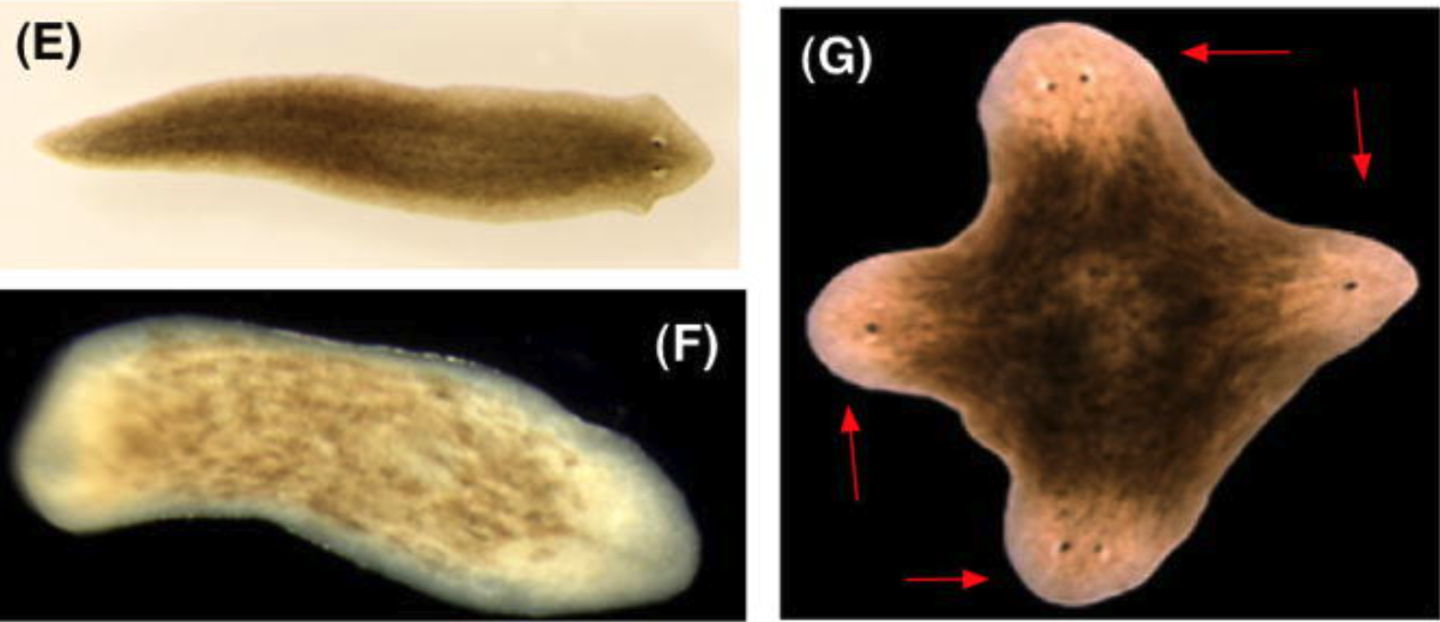
"Think about this – the pattern memory to which these animals will regenerate after damage has been permanently rewritten. And in fact, we can rewrite it back, and send them back to being one-headed without any genomic editing. This right here is telling you that the information structure that tells these worms how many heads they're supposed to have is not directly in the genome, it's in this additional bioelectric layer.
"Probably many other things are as well, and we now have the ability to rewrite it. And that, of course, is the key definition of memory. It has to be long-term stable, and it has to be rewritable. And we're now beginning to crack this morphogenetic code to ask how is it these tissues store a map of what to do, and how can we go in and rewrite that map to new outcomes?"
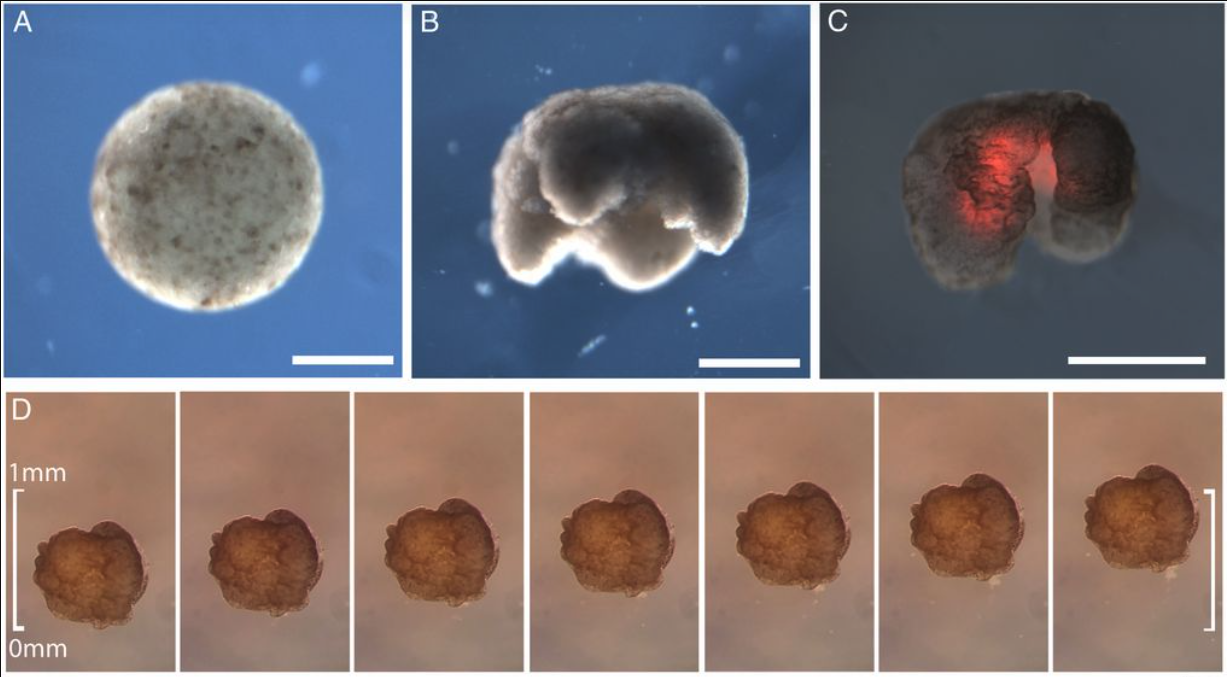
A quick note on Xenobots
In the interests of brevity, we'll skip over some of the other incredible work Levin and his collaborators have done on 'Xenobots' – freeing groups of cells from their organisms and allowing them to self-assemble into entirely new creatures, which use their cellular intelligence and bioelectrical communication to develop their own unique behaviors – or can be externally designed and programmed.
You can learn more about these remarkable living co-operatives around the 15-minute mark in the TED interview with Chris Anderson below.
Regenerating organs via 'subroutine' shortcuts
Yet another stunning feature of the team's work has been to demonstrate that hacking various organisms using this approach may actually end up being a lot easier than it might seem. Essentially you don't need to understand how to build a hand, or an eye, or a brain at a granular level; the cellular intelligence knows what to do, you just need to trigger the construction of the organ at a macro level.
Levin uses the example of building complete eyes, in the guts of tadpoles. "By triggering eye-building subroutines in the physiological software of the body," he tells Andersen, " you can very, very easily tell it to build a complex organ. This is important for biomedicine, because we don't know how to micro-manage the construction of an eye.
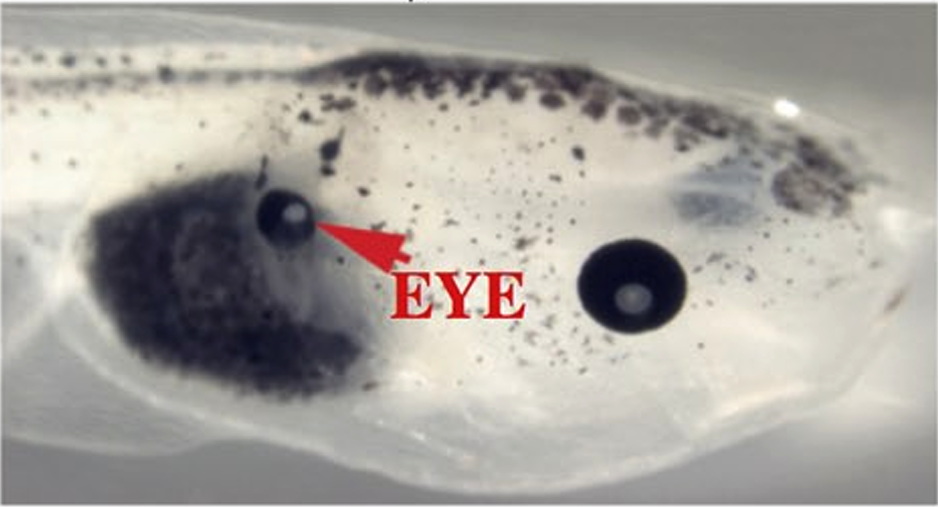
"I think it's gonna be a really long time before we can bottom-up build things like eyes or hands or so on. But we don't need to, because the body already knows how to do it. There are these subroutines that can be triggered by specific electrical patterns that we can find. This is what we call cracking the bioelectric code. And we can make eyes, we can make extra limbs... We can make extra hearts... We're starting to crack the code to understand where the subroutines are in this software that we can trigger."
"For example," he tells Maynard, "we can show that in adult frogs, which normally don't regenerate their legs, 24 hours of stimulation with a particular treatment that we came up with gives you a year and a half of leg growth. After that, we don't touch it at all... The idea is not to micro-manage the process, the idea is to convince the cells that this is what they want to do – and they have all the competencies about how to do it."
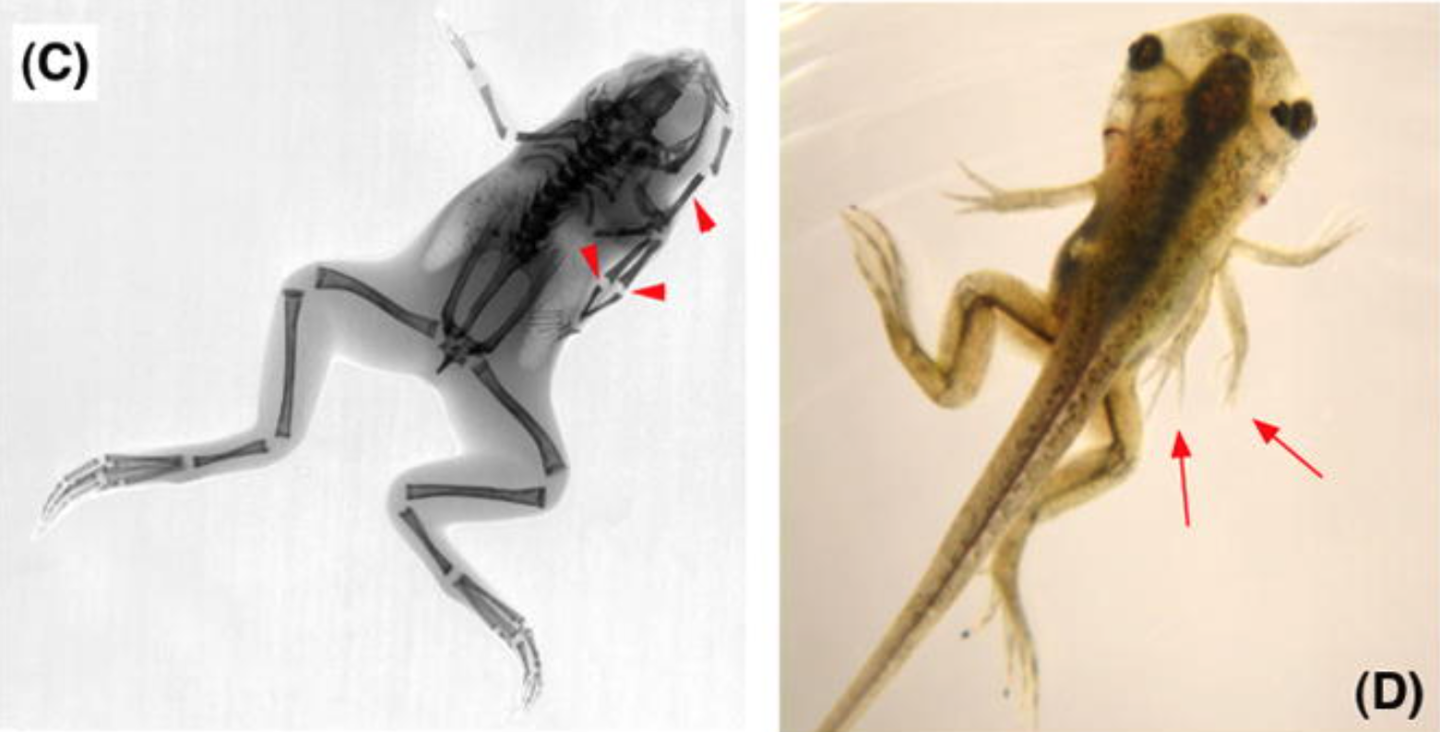
Revolutionary implications in regenerative medicine
If you can regrow whatever body part you like from scratch... Well, you've got an entirely new form of medicine with some flat-out spooky powers. Levin believes the potential for this work in cellular intelligence and bioelectrical hacking is profound.
"If you think about it," Levin tells Andersen, "most of the problems of biomedicine – so birth defects, degenerative disease, aging, traumatic injury, even cancer – they boil down to one thing: cells are not building what you want them to build. So if we understood how to communicate with these cellular collectives, and rewrite their target morphologies, we would be able to normalize tumors, we'd be able to repair birth defects, induce regeneration of limbs and other organs.
"These are things we've already done in frog models. The next really exciting step is to take this into mammalian cells."
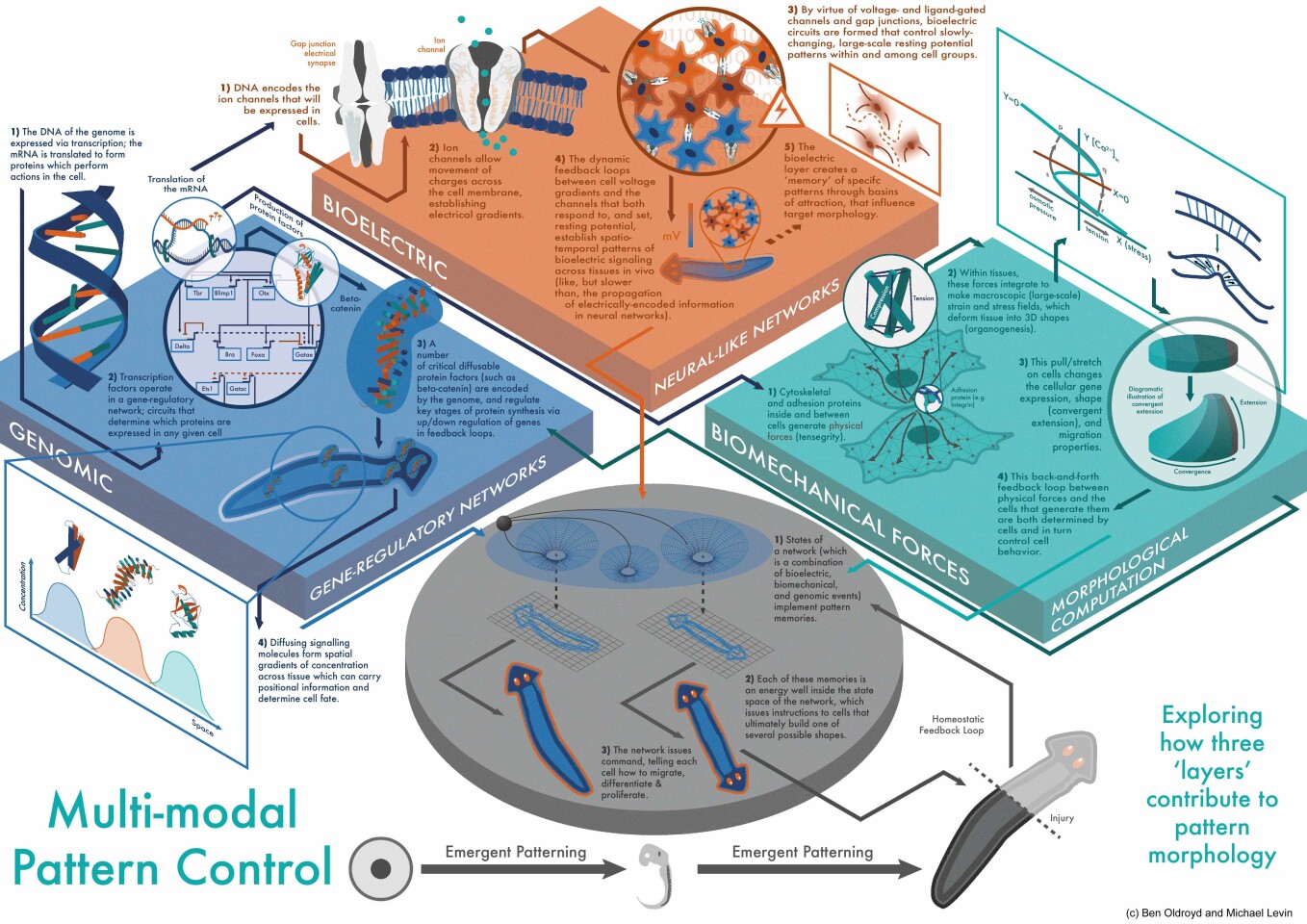
It goes deeper: Molecular intelligence
Levin's team has broadened its focus beyond just looking at living cells, finding that the subroutine patterns that can get part of a job done apparently nest all the way down to the molecular level.
"All of these levels," he tells Maynard, "are made up of sub-agents that solve problems in various spaces; anatomical space, physiological space, whatever – and they have different competencies and different agendas. Each layer is taking advantage of what I call this agential material – you have to engineer it very differently than you would engineer passive, or even active matter.
"And it goes even below cells. I mean, we're studying the learning capacities of molecular networks. Never mind whole cells, even the molecular networks have probably at least six different kinds of learning capacity.
"We need to have some kind of way of talking about molecular systems as having intelligence, because we have to be able to tell a story of scaling. We all start life as an unfertilized ova, a little blob of chemistry and physics. And there's no lightning bolt that at some point says 'ok, you were physics, but now you're a real mind.' We need a paradigm for how intelligence scales from simpler forms.
"The kind of intelligence that I'm talking about is the kind that William James defined as same goal by different means. So it's really a navigational intelligence. It's a publicly observable, perfectly empirically testable, problem-solving capacity. I am not talking about consciousness, I am not talking about self-aware meta-intelligence where you know how intelligent you are, I'm not talking about any of that. I'm talking about the ability to navigate a problem space and get your goals met, despite various new things that are going to happen."
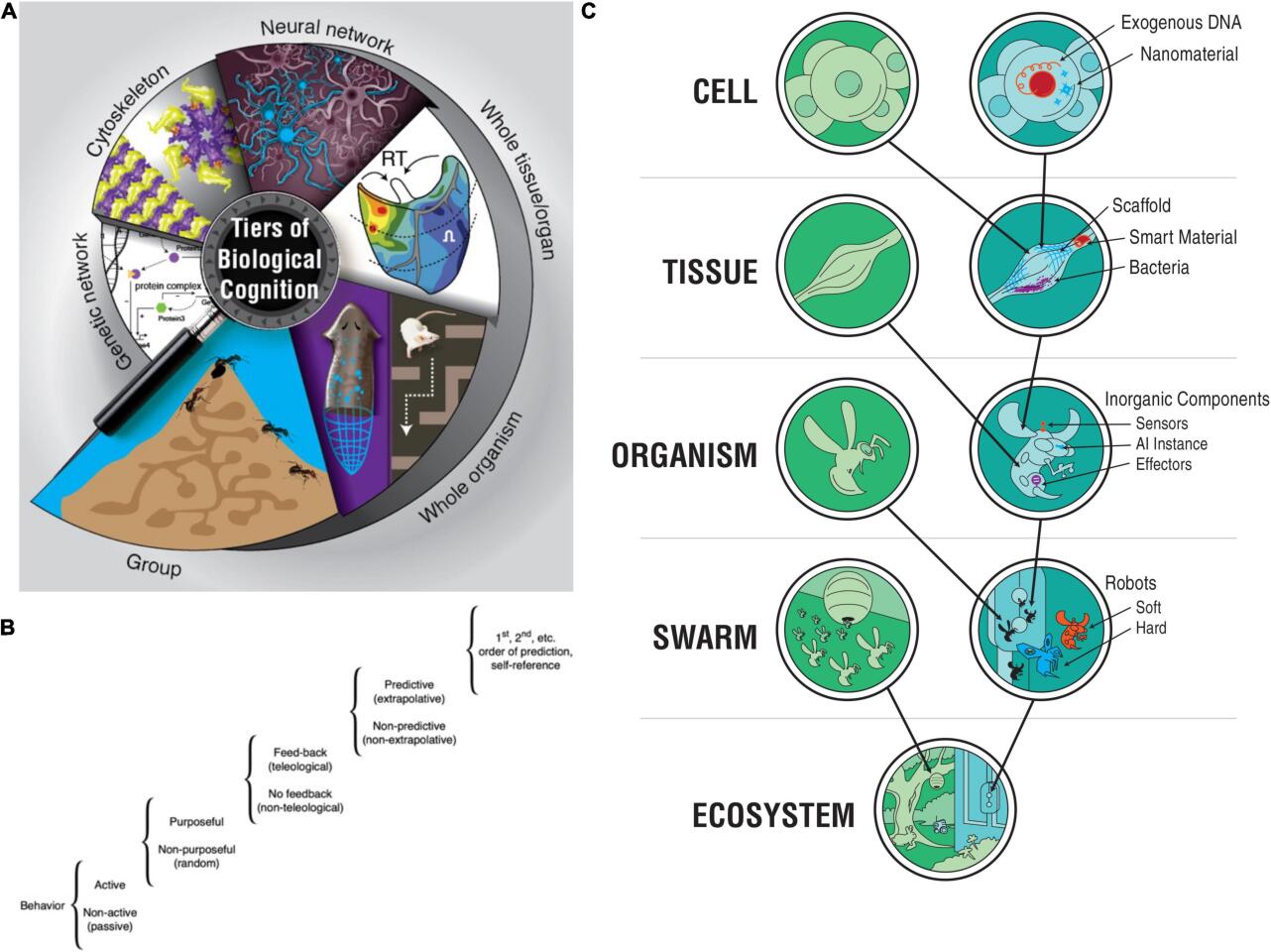
TAME: Technological Approach to Mind Everywhere
Levin and his team are starting to find testable examples of flexible, problem-solving 'intelligence' in so many places that they've had to nail down an approach to make sure they're not seeing ghosts in the machine.
"Two things about intelligence claims," he tells Maynard. "First of all, it isn't a philosophical claim. It's an empirical, testable, experimental claim. If you think some system has some kind of intelligence, you make a hypothesis about a problem space, about the goal it has, about the competencies you think it has, and you're going to do perturbative experiments.
"It's not anything goes, we don't paint hopes and dreams on rocks, we have very specific hypotheses about problem-solving capacities. You can't just imagine a spirit under every rock. But at the same time, you can't just assume cells don't have it.
"The other piece of that is, when you make a claim about the intelligence of some system, you're basically taking an IQ test yourself, because what you're saying as an observer is 'here is what I have noticed this system can do.' That doesn't mean you didn't miss a whole bunch of other things."
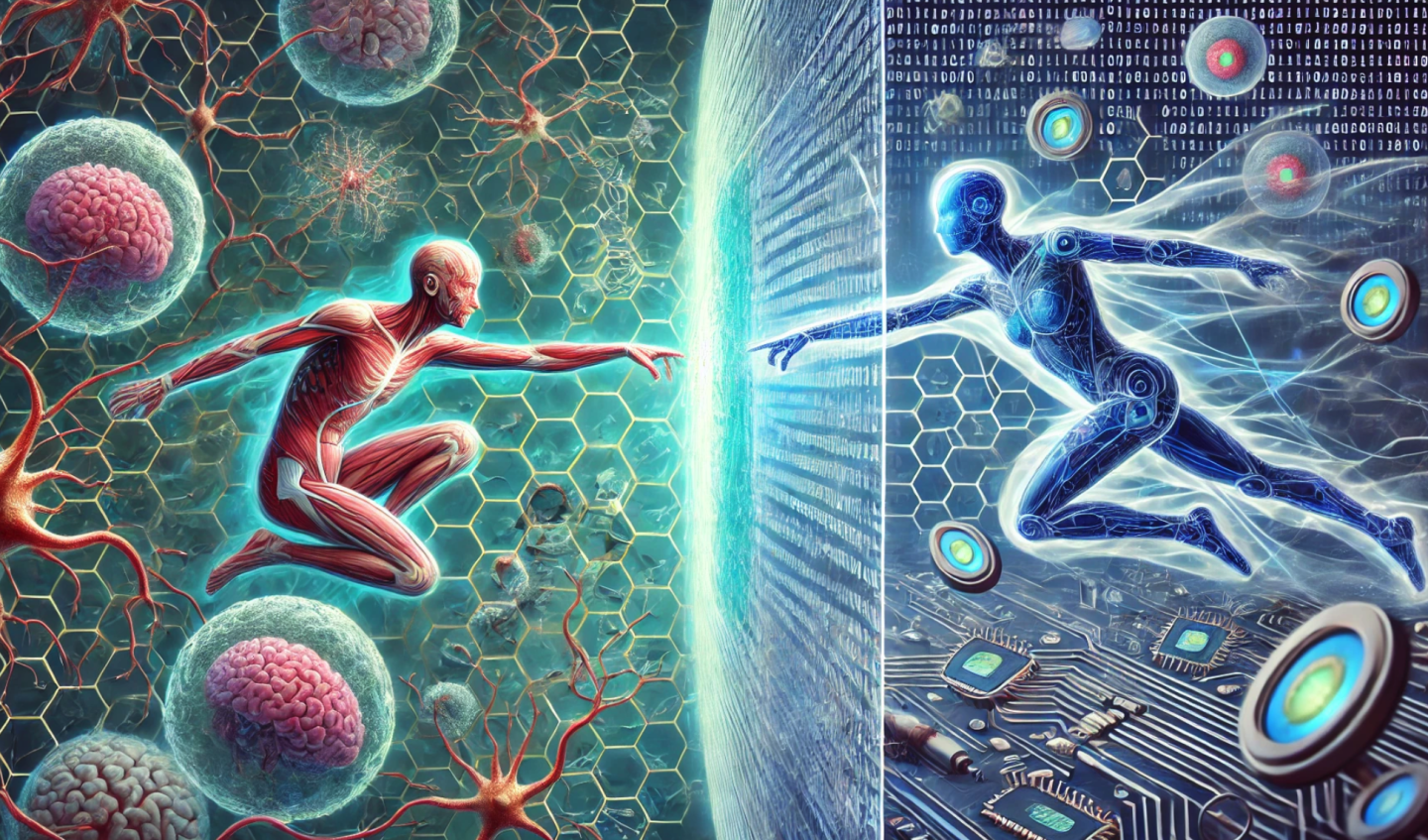
Consciousness and Artificial Intelligence: Where to draw the line
As with many cutting-edge areas in today's wild world of science and technology, Levin's work butts up uncomfortably close to the barrier of the unknown and the realms of philosophy or religion. Indeed, when it comes to artificial intelligence, in which researchers are struggling to understand exactly what separates a sufficiently advanced AI from a conscious being, you could almost say that Levin's work is approaching the same barrier from the opposite side.
Maynard asks how Levin's TAME framework might judge recent advances in AI and machine learning. "We can have some arguments about consciousness and so on," he replies, "but I don't think there's any way to argue that we don't have machines that have a considerable – in some cases, a human-level degree of operational intelligence."
Indeed in one paper published in the journal Frontiers in Systems Neuroscience, Levin argues for "a more inclusive framework of cognition."
"Whatever the differences between us and some kind of future AI architecture," he tells Maynard, "the answer's not going to be what people often say. Here are some bad answers: 'that's just a machine, it operates on the laws of physics and chemistry.' Well guess what, so do you. 'I know what it is, because it's linear algebra, and I built it.' But we find learning and memory in systems as simple as a few genes that turn each other on and off. A network of differential equations that represent genes turning each other on and off – never mind the whole cell, never mind the whole genome – can already do Pavlovian conditioning.
"And we've found unexpected problem solving capacities and behaviors in something as dumb as a sorting algorithm. Like bubble sort, that kind of selection. These things are deterministic; six lines of code, there's nowhere to hide, there's no magic. But if you look at them the right way, you find things you didn't know they could do. Things that are literally not in the algorithm. That tells me we need to have a lot of humility about saying we know what something does, or what it's capable of."
Indeed, Levin has decided to draw his own line in the sand between living cellular intelligence and artificial intelligence.
"A few months ago," he tells Maynard, "I started writing a paper to lay out very clearly, what are the half-dozen things about biology that are really critical for making true agents that matter in a moral sense. Like 'here's what biology is doing that none of our computer architectures are doing.'
"And I stopped. And I'm not going to write that paper. Not that it'll help – somebody else will do it eventually. But I don't want to be responsible for this stuff. To whatever extent I'm right, in having found some of the key features I think make for true sentient beings that we're going to need to take care of... To that extent, I don't want to be responsible for creating trillions of them, and having no control over how they're treated."
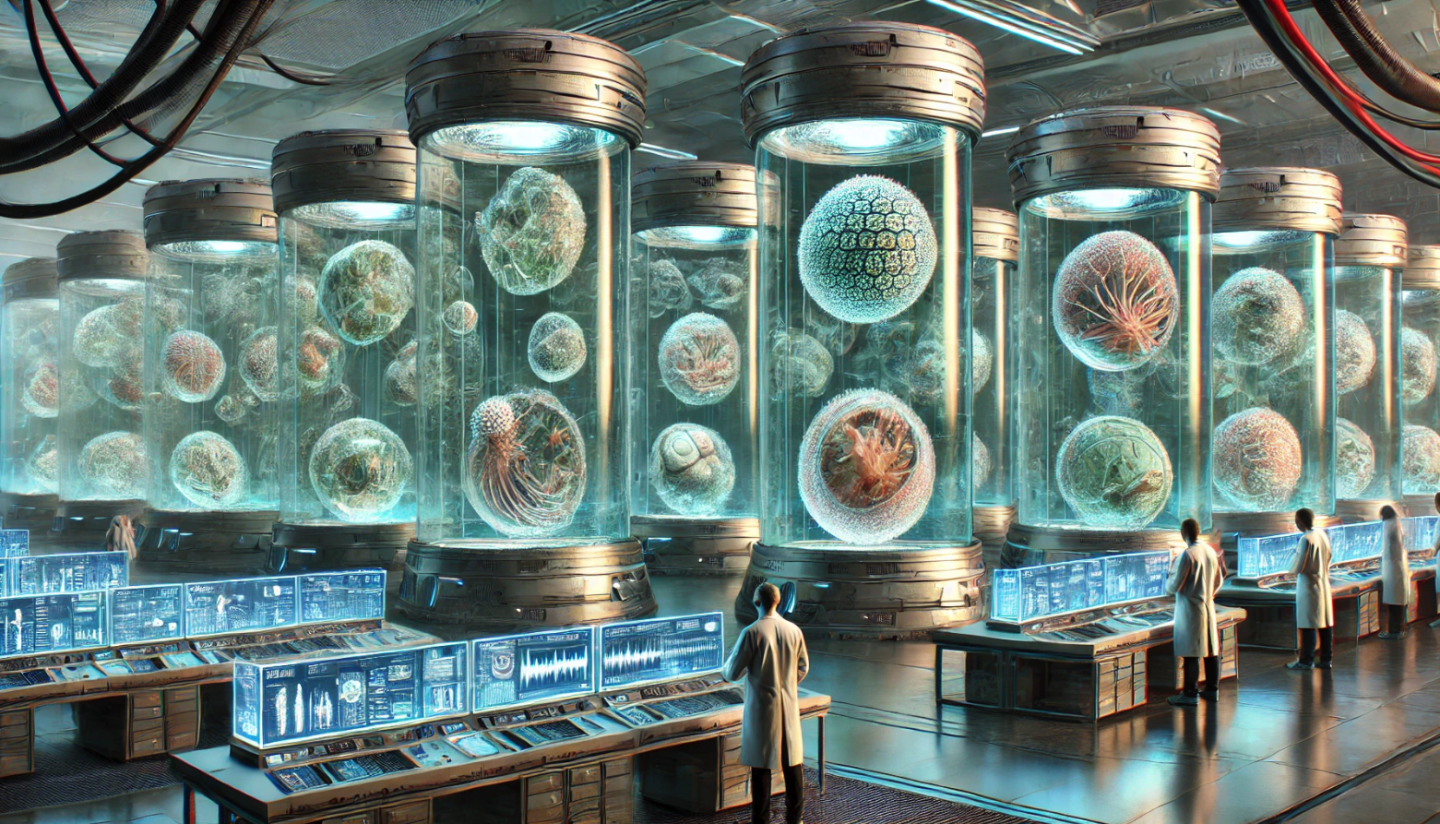
New forms of sentient life?
And that's where we wind up at this point in Levin's scientific journey: musing over whether it's possible to create new forms of conscious, intelligent life using the incredible insights and techniques he and his colleagues in this remarkable field have developed. And Levin is unequivocal.
"I think it's absolutely possible," he tells Maynard. "The idea that what's special about minds can only be produced by a blind, tinkering agent that makes mutations and selects certain things? I don't see why that process would have a monopoly on creating real minds.
"I know there are people that disagree on that. Richard Watson, I think, is one. But to me, I think there are many roads to doing this. I think we already have a good basis for figuring out what are the actual policies and components that are necessary. And they have nothing to do with being made out of protoplasm, or any of the things we assume are tied to the biology. I absolutely think they can be instantiated in other media.
"When I give talks about this stuff to a generic, random audience in conventional fields, most of this is things they've never heard of, or that sound completely wrong to them on a philosophical level. So I'm not sure where we are in that transition from 'this is impossible' to 'this is completely obvious.' I think that's the journey we're on, I'm just not sure where we are... Assuming we all live long enough, this is going to overturn everything. And it should."
So what, Maynard prompts, does this all mean for the future of being human? "The future is freedom of embodiment," replies Levin. "In the future I see, our children who are told stories of the past say 'you've got to be kidding me. You mean somebody was born, and just because of the vagaries of some cosmic ray hitting their DNA, they had to stay and die in the body they were born with? Maybe their goal required more IQ or a longer lifespan, but no, they got lower back pain and astigmatism and then died at 70? That can't be right. Nobody can live like that!'
"That's the future I see, that where we are now becomes ridiculous. And it should be. It is ridiculous."
Incredible stuff, and some of the most seductive and thought-provoking ideas I've come across in more than 17 years at New Atlas. Enjoy Levin's full April 2024 conversation with Future of Being Human Initiative Director Andrew Maynard below.
Sources: TED, The Future of Being Human, Michael Levin