A new approach for simulating the birth and evolution of galaxies and cosmic filaments within the Universe has been developed by researchers at the Harvard-Smithsonian Center for Astrophysics together with their colleagues at the Heidelberg Institute for Theoretical Studies. It is called AREPO, and has been used to simulate the evolution of our Universe from only 380,000 years after the Big Bang to the present. The full variety of spiral, elliptical, peculiar, and dwarf galaxies appear in the simulated Universe.
Scientists have been using computers to simulate the evolution of the Universe for about two decades. The cosmological model used for most simulations is the Lambda Cold Dark Matter (LCDM) model, which is considered the standard model for Big Bang cosmology. The model includes general relativity with a cosmological constant, and a predominant portion of dark matter and energy. It successfully describes the existence and structure of the cosmic microwave background, the large scale structure in the distribution of galaxies, the abundances of the light elements, and the accelerating expansion of the Universe. However, hitching the LCDM model to a 14 billion year simulation leading to the present of our Universe is not a trivial task.
The quality of such simulations has increased rapidly, owing as much to refinement of the simulation techniques as to the titanic increase in computational power over the past 20 years. High-accuracy simulations of the behavior of dark matter and energy in the LCDM model have been carried out. However, these are relatively simple simulations in that dark matter and energy are only thought to interact through their gravity. Including normal matter and energy is considerably more complex, because they do interact in ways that are important influences on the evolution of the Universe.
To model the formation of galactic structure requires treatment of many physical phenomena beyond that of gravity, including the effects of magnetism, hydrodynamics, radiation, multiple types of matter and energy, and shock waves among others. In most cases our knowledge of the physics underlying these phenomena is sufficient, but how to best simulate their mutual interactions on a computer is still being improved.
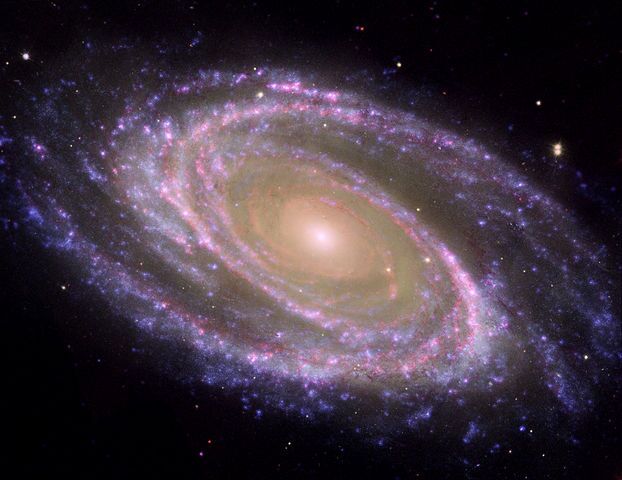
A glaring example is offered by galactic structure. For example, our cosmic neighborhood is full of large spiral galaxies like our Milky Way, and the Andromeda galaxy (M31). Such spirals are common, but cosmological evolution simulations do not generally produce universes containing large spiral galaxies. Rather they produce clumps of matter making up roughly spherical amorphous galaxies without anything like the broad disks and extended arms of a typical spiral galaxy. This is shown clearly in the following video where the gas distribution is shown for a galaxy from a cosmological simulation using the codes GADGET (bottom) and AREPO (top). The nested views show similar large scale structure (left panel), but reveal very different gas distributions around galaxies (right panel).
Two basic classes of simulation methods have been used to simulate the evolution of the Universe. These are Lagrangian Smoothed Particle Hydrodynamics (SPH) and Eulerian mesh-based hydrodynamics with Adaptive Mesh Refinement (AMR). Both of these methods have serious limitations for handling such complex physics. SPH treats shock waves and discontinuities poorly (a great deal of structure evolution takes place through collision of smaller chunks of matter), and suppresses the hydrodynamic instabilities which are largely responsible for growth of rotation in interacting bodies. The GADGET code referred to above is an SPH code.
AMR also has significant limitations, despite a long history in simulating hydrodynamics in many real world problems. They violate Galilean-invariance, which simply means that the results change if a massive body is moving across spatial coordinates instead of being at a constant position. This is not how physics works. The physical result should not depend on how an observer moves. For the same reason, a highly structured moving body will tend to lose its structure simply as a result of its motion in the simulation. Particularly unwelcome behavior is associated with AMR simulation of matter flows where the kinetic energy is large compared to the thermal energy: the usual situation in galactic evolution. This sort of non-physical prediction hampers one's trust in AMR simulation.
AREPO was designed to combine the best parts of SPH and AMR simulation methods while leaving behind the troublesome parts. The basic difference is in the spatial model for the normal and dark matter and energy in the Universe. The initial condition is split up into an unstructured set of smaller volumes by a mesh. AREPO uses a mesh that flexes and moves in space to match the motions of the underlying gas, stars, dark matter, and dark energy. The AREPO mesh can continuously and consistently adjust to local conditions to insure proper treatment of physics associated with normal matter and energy. AREPO retains the benefits of the SPH approach while avoiding most of the inaccuracies. It also retains the high-accuracy treatment of shocks, shear waves, and fluid instabilities characteristic of AMR. The devil is in the details, but the simulation approach used in AREPO is a great advance over previous methods.
The AREPO simulation of the Universe ran on Harvard's Odyssey high-performance supercomputer, using in total 1024 processor cores. The only information used as input to the LCDM model was the distribution of the 3K background radiation. Fourteen billion years was compressed into only a few months, leading to the video below, which gives a tour through the the last ten billion years of the evolution of the Universe ... or at least of a universe.
Source: Harvard-Smithsonian Center for Astrophysics