As the late Carl Sagan said, "we are made of star stuff," referring to the elements formed inside collapsing stars and disseminated throughout the universe in a massive explosion known as a supernova. Our knowledge of such events is set to expand, as for the first time an international team of scientists has captured the initial few minutes of a pair of supernovae as they exploded, as well as the first recorded supernova shockwave.
When a star the size of the Sun reaches the end of its life, the hydrogen fuel that powers its fusion reaction begins to run out. As time goes on, the core of the star fill with an "ash" of carbon until the core collapses, the outer shell of gas expands away, and all that is left is a white dwarf star of extremely dense solid matter.
However, if the star is between eight and 40 or 50 times the mass of the Sun, then it's a different story. Such massive stars are able to generate and sustain temperatures and pressures that don't just fuse hydrogen and helium, but heavier elements up to the weight of iron as well. Put simply, such stars develop into a mixture of different elements with a growing nickel-iron core.
So far, so routine, but the frightening thing is that as such stars age they have the potential to turn into what are called Type II supernovae. If the nickel-iron core at the heart of the star exceeds 1.4 solar masses, the forces that keep the star stable collapse suddenly and dramatically. The core collapses into a neutron star and the outer shell of gases rush in after it at up to 23 percent the speed of light. This produces temperatures of up to 100 billion kelvin and, to make a long story short, in a matter of minutes the star detonates in an explosion that can outshine an entire galaxy for weeks.
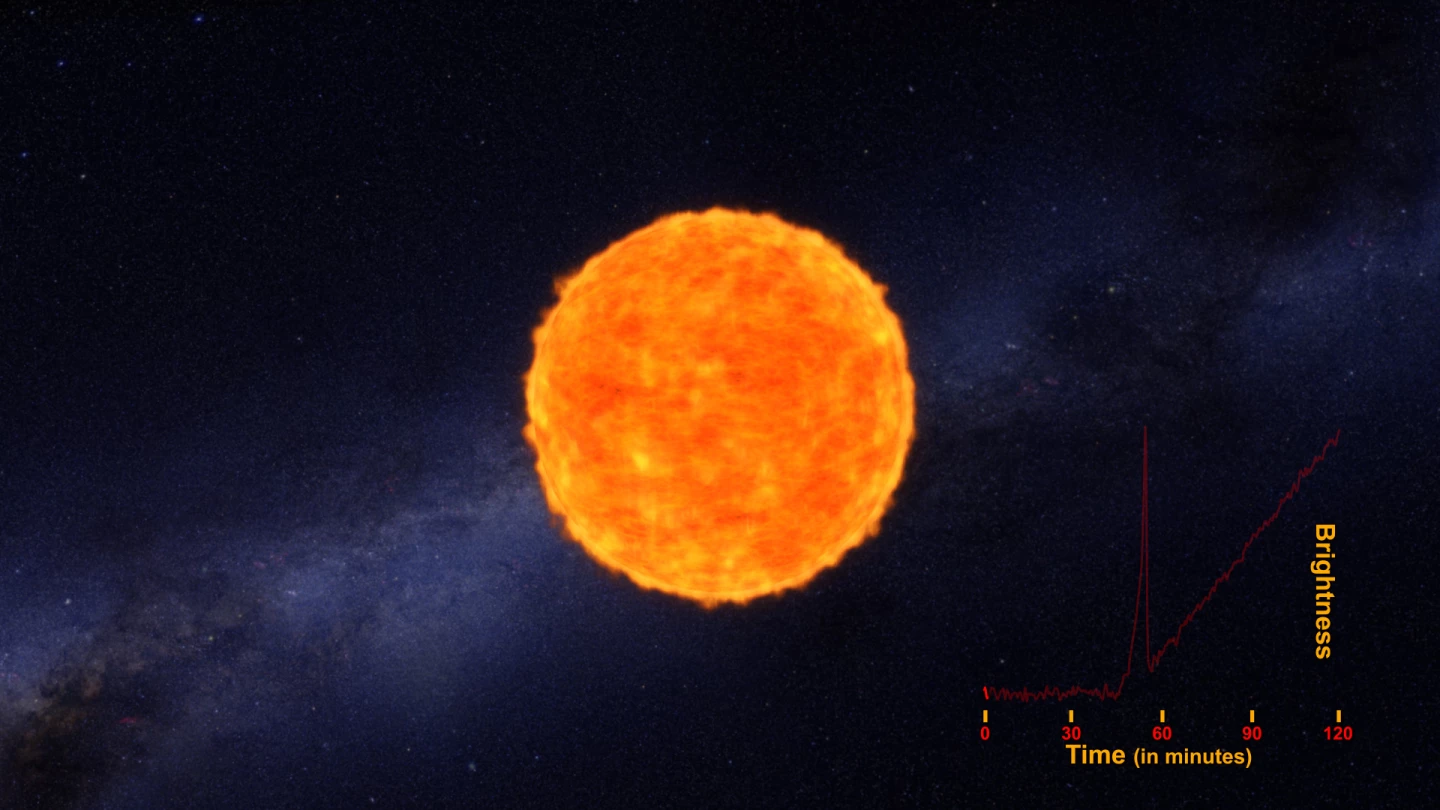
In itself, such an event is the very definition of impressive, but it's also very useful for astronomers, who use supernovae in distant galaxies as yardsticks to measure the universe. Even more importantly, the nuclear forces unleashed by a supernova explosion produce a massive shockwave that is responsible for the fusion reactions that create heavy elements, such as gold, silver and uranium.
The problem is, not much is known about exactly what goes on in the early stages of a supernova. For example, it's known that neutrinos transfer the energy from the collapse that produce the shockwave of the explosion, but exactly how is still a mystery.
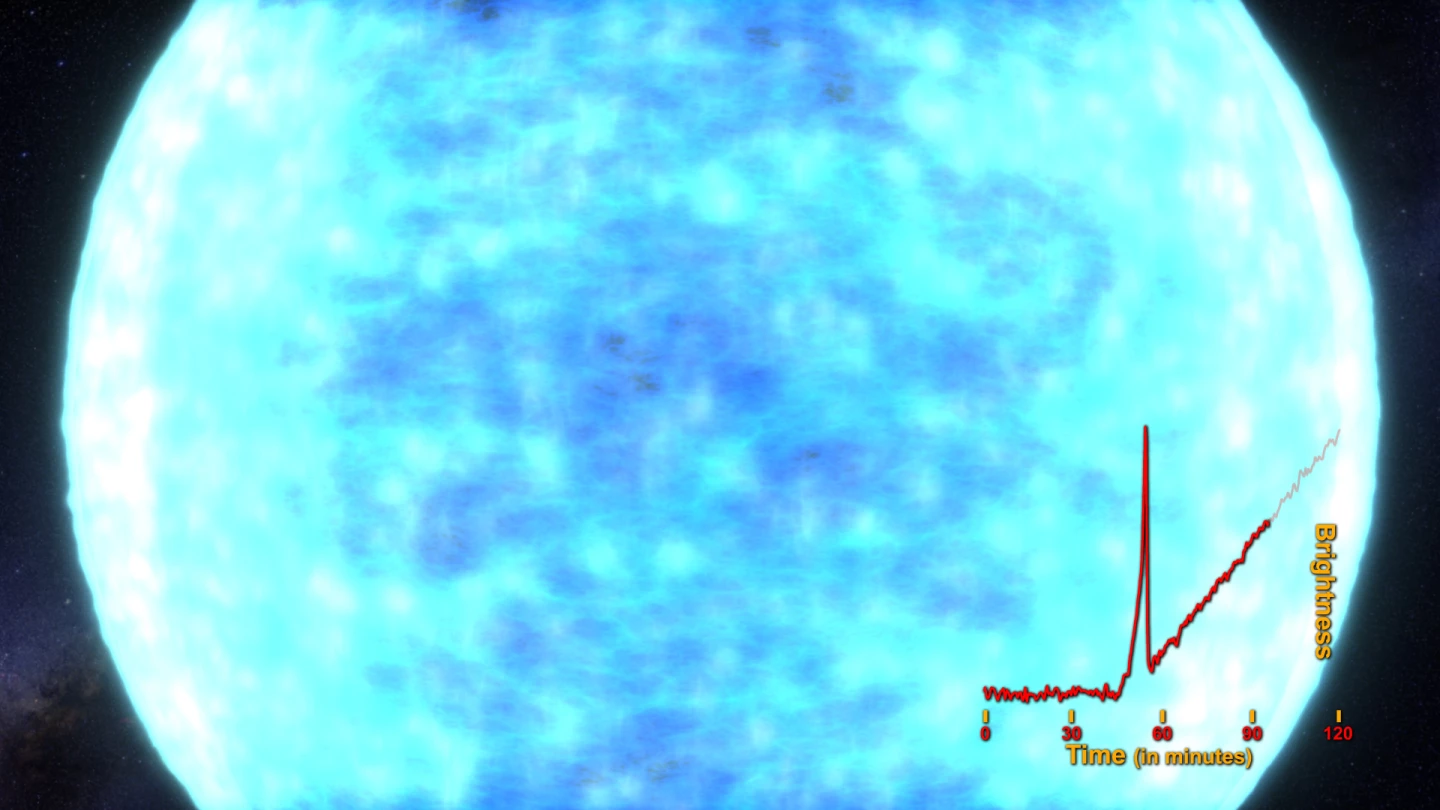
Using data from the Kepler Space Telescope, the astronomical team, made up of scientists from the Australian National University (ANU), the University of Notre Dame, the Space Telescope Science Institute (STSci), the University of California Berkeley, and the University of Maryland, observed two supernovae in two separate, distant galaxies as they detonated. One was 270 solar masses and the other was 460 solar masses, with both Type II-P supernovae. The P refers to the light curve, which plateaus after the explosion rather than falling off.
What the astronomers saw was not just the stars in the first minutes of an explosion of galactic proportions, but in the smaller of the two the shockwave of the blast could be seen, even though it is certain to have existed. According to Dr Brad Tucker of the ANU Research School of Astronomy and Astrophysics, this shockwave could not be seen in the larger because of the star's size.
"The star was so large that the shockwave did not travel all the way to the surface," he said.
The team says this fact new data will help further our understanding of theeffects a star's size and composition has on the early moments of its explosivedeath.
The team's findings were published in the Astrophysical Journal.
The animation below from NASA/STSci shows a supernova explosion.
Source: Australian National University