An international team of scientists using one of the pair of 10-meter telescopes at the W.M. Keck Observatory on the summit of Mauna Kea on the island of Hawaii has shown that the early moments of our Universe closely followed the theory for how elements formed shortly following the Big Bang. Improvements in how early elements are examined and in models of stellar atmospheres show that, contrary to previous studies, the kinds and quantities of atoms that made up our early Universe agree with the predictions of the Big Bang model.
In the Big Bang model, the elements were formed during the first 20 minutes of the life of the Universe. Formed during this period were the hydrogen isotopes hydrogen and deuterium, the helium elements He-3 and He-4, and the lithium elements Li-6 and Li-7. All heavier elements were formed much later, in the burning of the first generation of stars and as those stars became supernovae.
The Big Bang model predicts how many atoms of various kinds made up the early Universe. About 75 percent of the normal matter (as opposed to dark matter) in the early Universe was hydrogen, and about 25 percent was the more common form of helium having two protons and two neutrons in the nucleus.
The remaining elements were formed only in very small amounts. Deuterium (hydrogen with an extra neutron) and helium, with only one neutron each, form a few atoms out of every 100,000. There were also traces of two lithium isotopes formed. About three parts in 10 billion are Li-7 atoms (three protons and four neutrons) and seven parts in a million billion are Li-6 atoms, which have one fewer neutron than does Li-7.
Astronomers have been able to measure the relative amounts of these primordial isotopes using spectrographic studies of intergalactic gas and of very old stars. These studies have agreed nicely with the Big Bang predictions for the amounts of the hydrogen and helium isotopes.
Historically, observation and the Big Bang model have not agreed concerning the amount of the lithium isotopes. The observations have rather consistently seen less Li-7 than expected, and hundreds of times more Li-6 than the unobservable trace predicted for the Big Bang. To cure these discrepancies within the general structure of a Big Bang model has proven a difficult task.
One may well ask where you point a telescope to look at the proportions of various isotopes in elemental abundances in the very early Universe. This is a tough problem, as the formation of the elements occurred long before the microwave background radiation flowed out into the Universe about 300,000 years after the Big Bang. As a result, direct observation of the time when elements were first formed is virtually impossible.
Astronomers instead measure the present composition of old and very metal-poor stars as a stand-in for the state of the early Universe. Save for tiny traces of lithium formed in the Big Bang, the metals (which, to astronomers, are all elements heavier than helium) in the present-day Universe all were formed during the life or death of a star. When a star has very few metals, it is thought likely (based on our knowledge of stellar evolution) that the material in the star mimics the composition of the early Universe. This is particularly true for the lithium isotopes, as these are rarely produced through nuclear fusion.
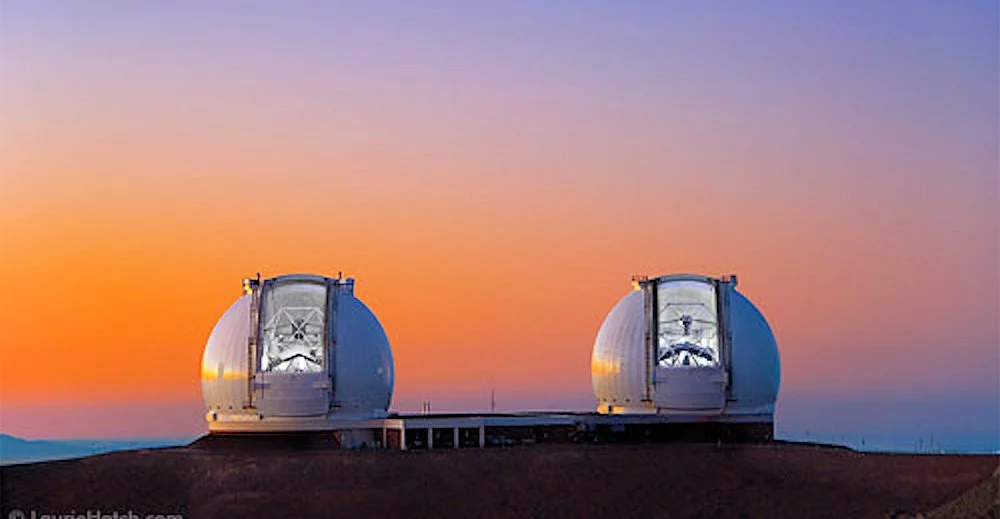
The Keck astronomers, led by Professor Karin Lind of the University of Copenhagen, used a high-resolution spectrograph on one of the Keck 10-meter telescopes to examine in detail the spectra of four extremely metal-poor stars (HD 19445, HD 84937, HD 140283, and G 64-12). One of these, HD 140283, has been called the Methuselah Star, and is nearly as old as the Universe itself. Whereas the Sun is about 2 percent metal, these target stars are roughly 0.1–0.01 percent.
Even with some 80 sq m (861 sq ft) of collecting surface, several hours of observation are required to acquire the spectra of these four stars. It isn't that the stars are dim – three of the four can be seen with a pair of binoculars. Instead, the lengthy exposures are needed because the light collected from the stars is spread out by the extremely high resolution of the Keck spectrograph, so the image of the spectral lines is very dim. This is a task that today could only be carried out be the Keck I telescope.
Interpretation of the stellar spectra is also difficult, particularly when it comes to lithium. The spectral lines for Li-6 are so close to those for Li-7 that they do not appear as separate. Instead, the Li-7 spectral lines seem distorted asymmetrically by the addition of the much smaller Li-6 lines. The problem is that local dynamic structures in the atmosphere of a star can also cause the Li-7 lines to appear asymmetric in the same manner. This is caused by convection cells (like solar granules) and other structures that are locally not in thermal equilibrium.
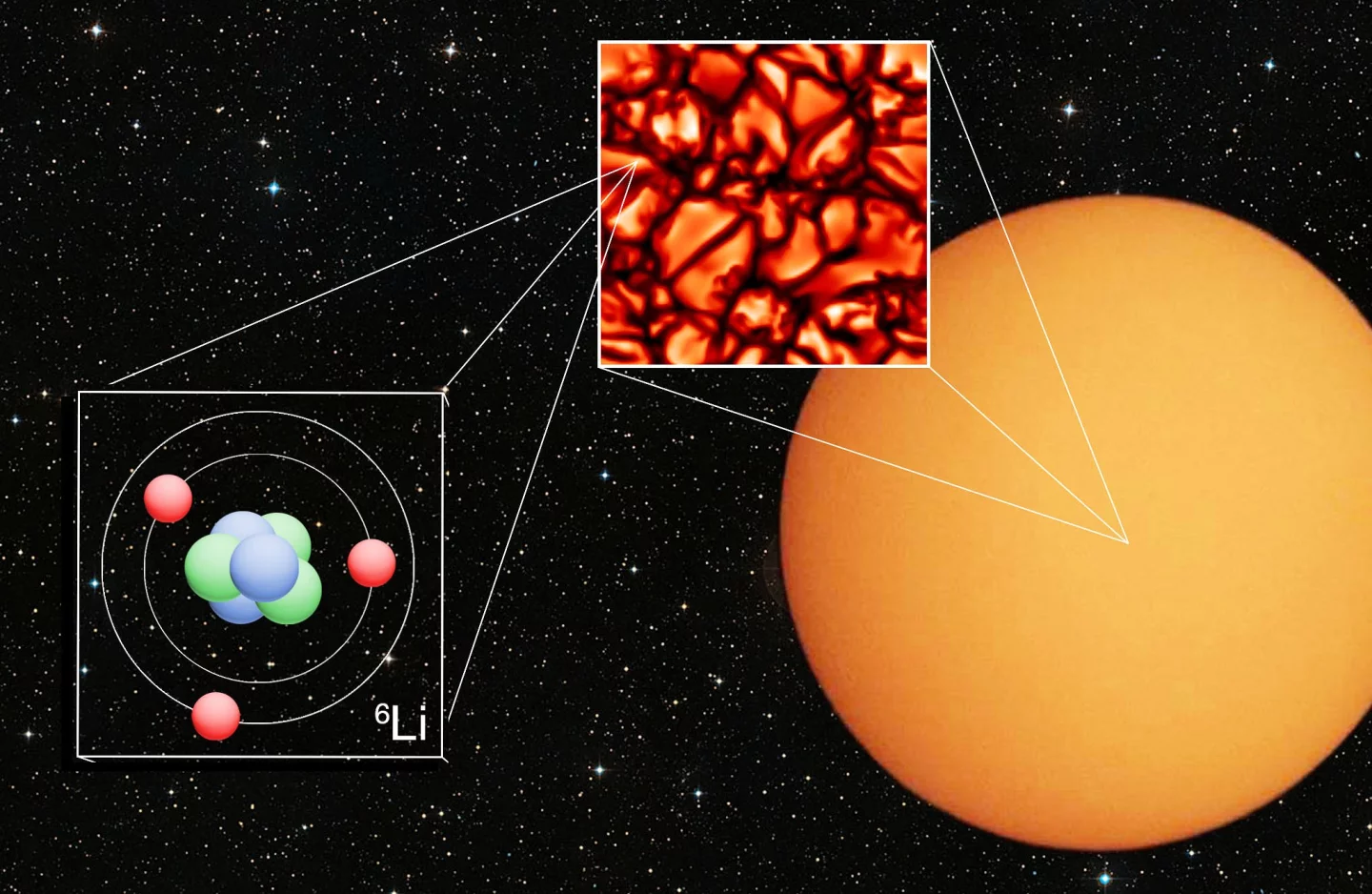
To properly evaluate the spectral lines, the constantly increasing computing power available to scientists now allows a full three-dimensional non-thermal-equilibrium model to be used to describe the various sorts of physics going on in the stellar atmospheres.
Prof. Lind's team found that the spectral asymmetries were actually caused by the dynamics of the stellar atmospheres, rather than by the presence of a large amount of Li-6. When compared to the new stellar models, the amount of Li-7 in the atmosphere of the stars studied rose enough to agree with the predictions of the Big Bang model.
“We simultaneously relaxed two key physical assumptions in the modeling of stellar atmospheres; one-dimensional hydrostatic and local thermodynamic equilibrium,” said Prof. Lind in a press release. “Using more sophisticated physics and powerful super-computers, we managed to remove the systematic biases that plague traditional modeling and have previously led to false identifications of the 6Li/7Li isotopic signature.”
Lind's team has largely set to rest the most glaring discrepancy between our analysis of what should happen during and after a Big Bang, and our observations of a Universe that, to all current indications, evolved out of something very like a Big Bang. As seems true of so much of modern physics and cosmology, simply because a description of some property of the Universe seems contrary to common sense doesn't mean the description isn't close to the truth.
The team's discovery is published in the June edition of the international journal Astronomy & Astrophysics.
Source: W.M. Keck Observatory