For nearly a century, scientists have been tantalized by the prospect of attaining an inexhaustible source of energy through nuclear fusion. Unfortunately, engineering a controlled environment where atomic nuclei can continuously fuse under extreme pressure and temperature to produce energy that we can capture is very difficult, but that doesn't mean exciting advances aren't being made. Here we take a look at some of the different approaches to nuclear fusion, and the reasons why some appear more promising than others.
Fusion and fission are different processes for producing nuclear energy. Where nuclear fusion seeks to combine separate atoms into a larger one, nuclear fission relies on breaking apart an atom (usually Uranium 235) by striking it with a neutron. Both processes release huge amounts of energy, though fusion produces more.
This energy produced by nuclear fission is captured inside reactors, like those at Fukushima and Chernobyl, and used to heat water into steam, which spins a turbine and generates electricity. But this process creates waste products that can remain radioactive for millions of years, which, as we have seen at Fukushima and Chernobyl, can become a disaster when things go awry.
Fusion, on the other hand, would produce no long-lasting nuclear waste, with the necessary materials able to be recycled within 100 years. There is also no danger of a meltdown or nuclear accident because it relies on high-temperature reactions that cool within seconds when disrupted. And because these reactions use relatively small amounts of fuel, there is no danger of them being harnessed to produce nuclear weapons.
The field of nuclear nuclear fusion research involves scientists solving all sorts of problems, but all are working towards the same goal, which is recreating the processes that the Sun itself uses to produce monumental amounts of energy. Huge gravitational forces confine hydrogen from the Sun's atmosphere and use intense heat and pressure to convert the gas to plasma, in which nuclei collide at high velocity to form helium and release energy.
"Solar power is really fusion power, just at a distance," Matthew Hole, a nuclear fusion expert and research fellow at Australian National University, tells New Atlas. "All this power is just fusion reactions coming from the Sun, that's all a solar array is doing from the edge of the reactor. But the reactor happens to be eight light minutes away."
Another key factor is gravity. The Sun's tremendous gravitational forces are around 28 times greater than here on Earth, which means we've had to get creative with the way we confine our fuel for nuclear fusion reactions. The favored approach as it stands is through the use of magnetic fields, which can be used to confine two heavy forms of hydrogen, deuterium and tritium, in a donut-shaped device called a tokamak.
Attack of the tokamaks
Tokamaks are one example of a magnetic confinement system for nuclear fusion, and is the one Hole considers the most feasible in terms of net power generation. These consist of a neat series of coils placed around a torus-shaped reactor, where a plasma is heated to millions of degrees with the help of a strong internal current. The idea is to hold the plasma in place for long enough for the fusion of nuclei to occur.
The first tokamaks were designed in the 1950s, and in 1991 the Joint European Torus (JET) tokamak in the UK became the first device to achieve a controlled release of fusion power. It then set a power output record for a tokamak device with a 16-MW effort in 1997. Despite the achievement, it still required 24 MW of power to heat the plasma, meaning the experiments fell well short of the net gain energy generation needed to make nuclear fusion feasible.
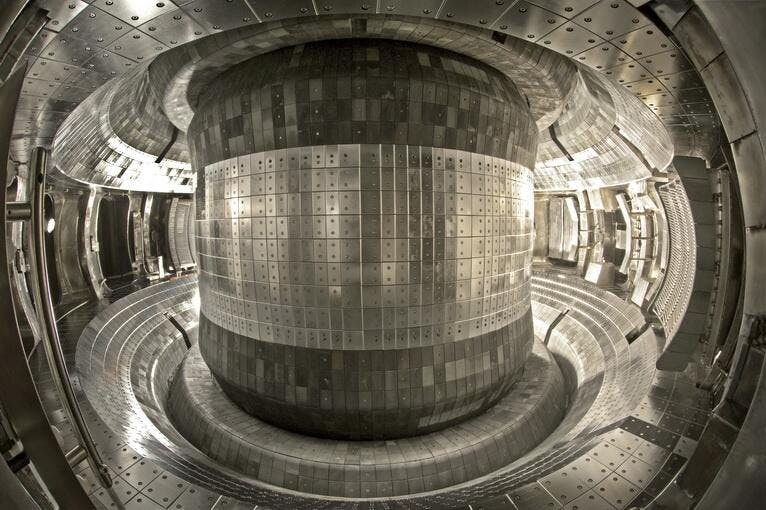
JET remains in operation today and has since been joined by a slew of other tokamak devices around the world. The list includes China's Experimental Advanced Superconducting Tokamak, which back in 2018 managed to hit temperatures of 100 million degrees Celsius, the Korea Superconducting Tokamak Advanced Research (KSTAR) device which last December set a world record by holding plasma at over 100 million °C (180 million °F) for 20 seconds, and private ventures like UK company Tokamak Energy.
Despite these achievements, the energy breakeven point for fusion remains elusive. For many working towards this goal, a next-generation device currently under construction carries much of their hopes. ITER, or the International Thermonuclear Experimental Reactor, is one of the most ambitious energy projects humankind has ever undertaken, involving scientists and engineers from 35 countries. It will become the world's largest nuclear fusion device when completed in 2025.
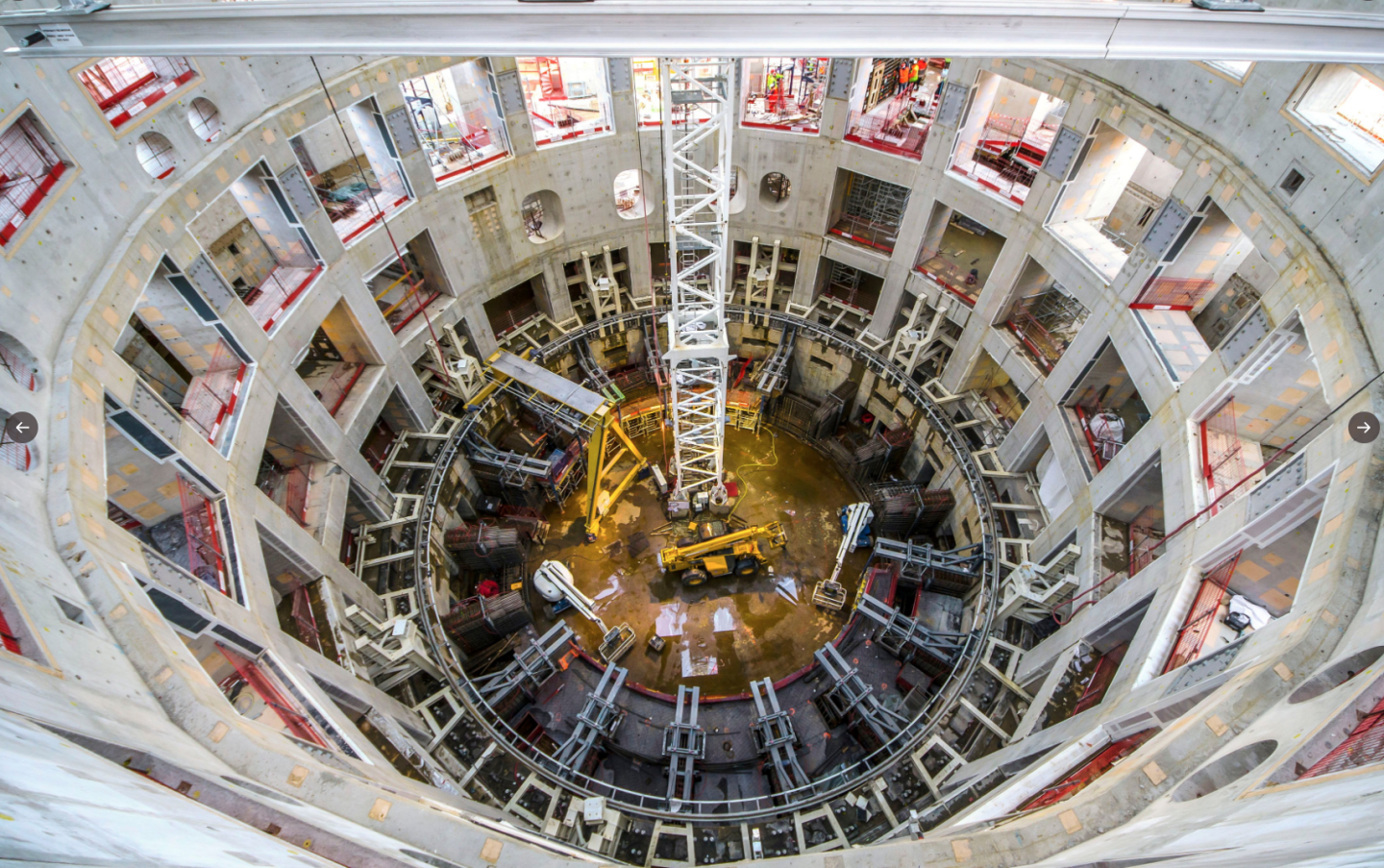
The seven-story tall ITER will be a tokamak, and will accommodate streams of plasma 10 times thicker than those forming in the tokamaks of today. Where JET managed to produce 16 MW of power output from 24 MW of power input, ITER is designed to produce 500 MW of power from a 50 MW input, finally demonstrating a net energy gain. This energy won't be captured as electricity. Rather, ITER will serve as a testbed for the technologies it is hoped will underpin the first ever fusion power plants.
While much of humankind's ambitions in nuclear fusion are tied up in ITER, there are other exciting possibilities in the mix, including another magnetic-confinement fusion reactor you could consider a twist on the tokamak: the stellarator.
Doing the twist - The Stellarator design
Like tokamaks, stellarators are designed to confine streams of plasma in a looping chamber with the help of magnetic coils, but with some key differences. Rather than a nice symmetrical doughnut shape, a stellarator sends its plasma around in irregular circles that twist and turn with an incredibly complex series of magnetic coils. It seems counterintuitive, but this actually creates more stability in the plasma owing to differences in the internal current, as Hole explains.
"In toroidal magnetic confinement, you need the current to twist," he says. "Tokamaks do this with large internal current that causes the field to twist and rotate as it goes around the bend. In a stellarator, you deliberately twist the whole cross section of the bend. With twisted coils, you twist the magnetic current. This means you don't need a large internal current to generate the twist. So in some sense, you're translating a physics problem into an engineering problem."
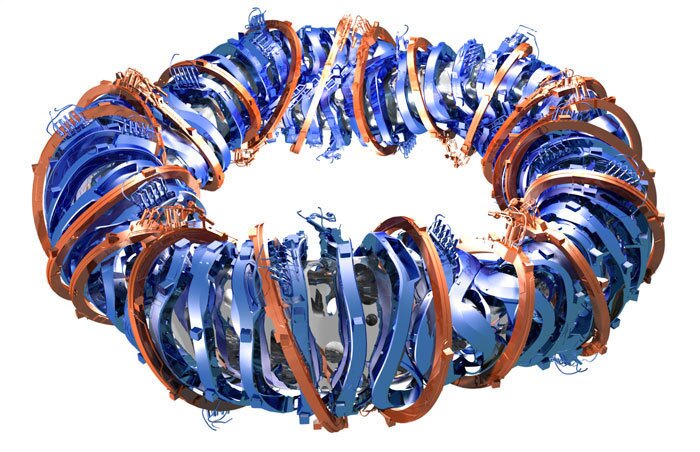
This also creates a huge mathematical problem to describe the twisting torus, Hole notes. And so far as mathematical and engineering problems go, they don't come much bigger than Wendelstein 7-X, the world's largest stellarator and one that couldn't be designed without the help of supercomputers. Built to continuously contain super-hot plasma for more than 30 minutes at a time, Wendelstein 7-X was fired up for the first time in 2015 and has since been taking baby steps toward that goal.
It progressed from confining helium plasma to hydrogen plasma in 2016, before these sub-second-long flashes became confinements lasting longer than 100 seconds in 2018, a record for a reactor of its type. These took place at temperatures hotter than the Sun, at around 20 million °C (36 million °F), with the scientists also achieving unprecedented energy yields.
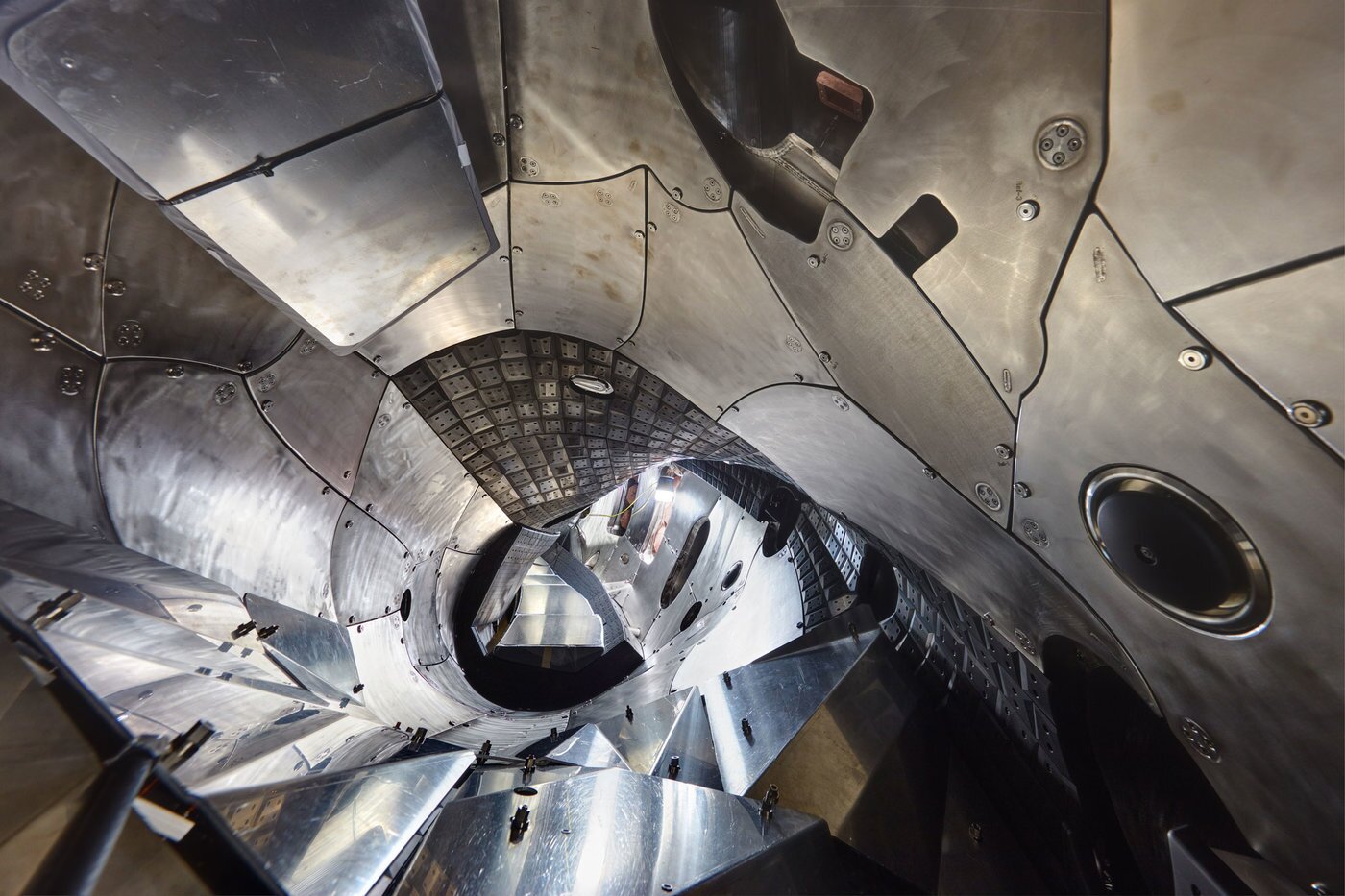
Because stellarators don't require such a large internal current and inherently offer more stability than tokamaks, Hole says they may well be better suited to providing power to the grid. But that's assuming the stupendously complex infrastructure can be built in a way that isn't stupendously expensive, and it can achieve the same confinement, which isn't the case as it stands.
"A stellarator has more intrinsic appeal, perhaps, than a tokamak, in the long term," Hole explains. "But to an engineer, a stellarator is a bit of a nightmare. So thats why both are worth exploring."
Doing away with the magnets
Beyond the realms of magnetic confinement, there are other approaches to nuclear fusion being explored, falling under what's known as inertial confinement. This newer arm of nuclear fusion research looks to use very precisely targeted laser or ion beams to rapidly heat up a pellet of fuel, which would again be made up of deuterium and tritium (generally speaking).
The idea is that subjecting these fuel pellets to such sudden and intense heat would cause tremendous compressive forces that trigger a chain reaction through the layers of material in which nuclear fusion can take place, releasing huge amounts of energy. Back in February, we looked at a radical approach to this from Australian company HB11 Energy, which seeks to do away with the conventional deuterium and tritium recipe in favor of a non-radioactive approach involving hydrogen and boron-b11.
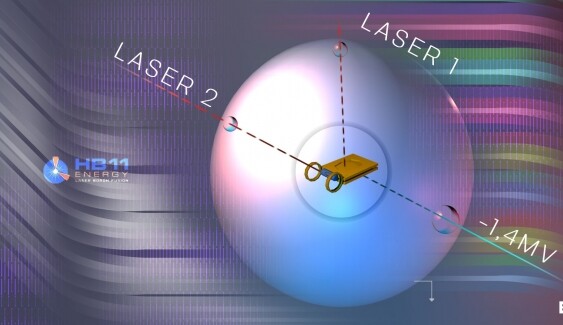
The company claims to be sidestepping many of the challenges that have plagued nuclear fusion power for decades, largely because it isn't trying to heat its fuel to insanely high temperatures. It subjects its fuel pellets to two lasers, one to establish a magnetic containment field and the second to trigger a chain reaction of hydrogen-boron fusion, which creates particles that in turn can generate an electrical current.
This current, the team says, can be almost directly channeled into an existing power grid. There'd be no need for a steam turbine generator or heat exchanger, and no danger of a meltdown. The team is very bullish about its technology and says its experiments are returning reaction rates a billion times better than predicted, and believe its development roadmap will be much faster and cheaper than other approaches.
"It is interesting science," says Hole, who is familiar with HB11 and the researchers working on the project. "But I wouldn't say there is credible evidence to suggest you could turn that into a power plant on a timescale faster than ITER or toroidal magnetic confinement. In my mind, there are even more challenges."
A key problem with these types of approaches, Hole says, is that these reactions take place in the blink of an eye. For the technology to be applied to a practical power plant, it would need to mature from short-lived, one-off reactions to something that produces a steady supply of energy, like a burning fire.
"If I fire a bunch of lasers at a target and the whole thing is over in a nanosecond, that is a pulsed experiment," he explains. "To repeat it, I put the target back in place and I put the wires back in place, because I blew the whole thing up, it is gone. The question is how do you translate something that is intrinsically pulsed into something that is intrinsically steady state? In the case of these experiments, you'd need to go from one pellet a week, to 10 pellets a second."
Feeling the pinch
Another interesting example of an inertial confinement approach to nuclear fusion is what's known as the Z-pinch. Rather than using large and complex magnetic coils to hold streams of plasma in place, the Z-pinch approach uses the electromagnetic field that is generated within the plasma itself. Z-pinch has been called the dark horse of the nuclear fusion race because, since its inception in the 1950s, it has promised a far simpler configuration than tokamaks or stellarators. But like those devices, it too has been prone to instabilities in the plasma, which escapes the magnetic field lines and forms problematic bulges.
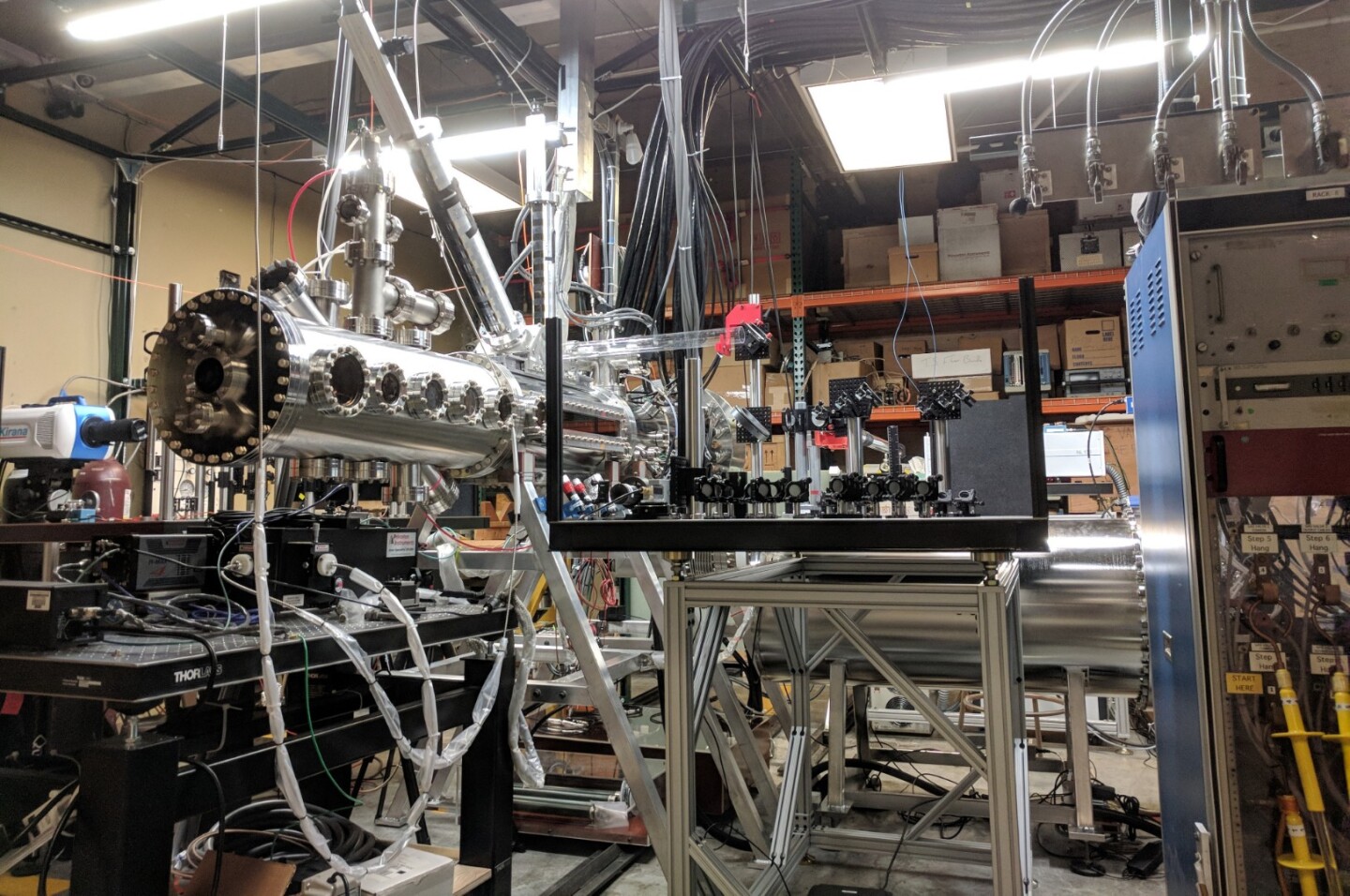
In 2019, we looked at research from a team at the University of Washington that came up with a way to smooth out these bulges by tweaking the fluid dynamics of the plasma. In a 50-cm-long (20-in) Z-pinch column, the team was able to hold their flowing plasma in place 5,000 times longer than static plasma, and observed energetic neutrons that they considered telltale signs of nuclear fusion. While promising, like the HB11's laser approach, Z-pinch is still going to run into grand challenges when it comes to providing continuous power.
"The Z-pinch is also intrinsically pulsed, they implode a set of wires," says Hole. "It's not going to be intrinsically steady state."
A fusion-powered future?
Hole says most of the concepts he has looked at for a nuclear fusion facility are based on the tokamak design, though there are others floating around based on the stellarator configuration. All eyes will be on ITER when it fires up for the first time, particularly as it commences experiments using a 50:50 mix of deuterium and tritium in 2025.
So as we can see, while ITER, toroidal magnetic confinement and the tokamak design are certainly the most progressed, nuclear fusion researchers are pursuing a number of different approaches, all with their advantages and disadvantages. Unfortunately, regardless of the approach, nuclear fusion still requires more energy from us than it gives back. But solving those engineering and physics problems to achieve a net gain in energy, incredibly complex as that will be, would be one of humankind's grandest achievements.
"None of these things are ideal, if it was ideal and simple, we would have already done it," Hole says. "It's a bit like putting man on the Moon, it's a very hard thing to do."