Particle physicists have been eagerly awaiting the first trials of the new Main Injector neutrino beam at the Fermi National Accelerator Laboratory in the US. This new facility is the result of reconfiguration of the Fermilab particle accelerators in the wake of the shutdown of the Tevatron in 2011. The new beam source is now online, and is well on route to becoming the world's most intense focused neutrino source.
A great deal of effort goes into studying neutrino physics and interactions, owing in large part to the indifference of neutrinos to the rest of the universe. Invisible to electromagnetic and strong interactions alike, a neutrino's only known external influences are via the weak interaction and gravity. A very large number of very well behaved neutrinos are required to enable experiments to study the interaction of neutrinos with matter, neutrino oscillations, and neutrino mixing.
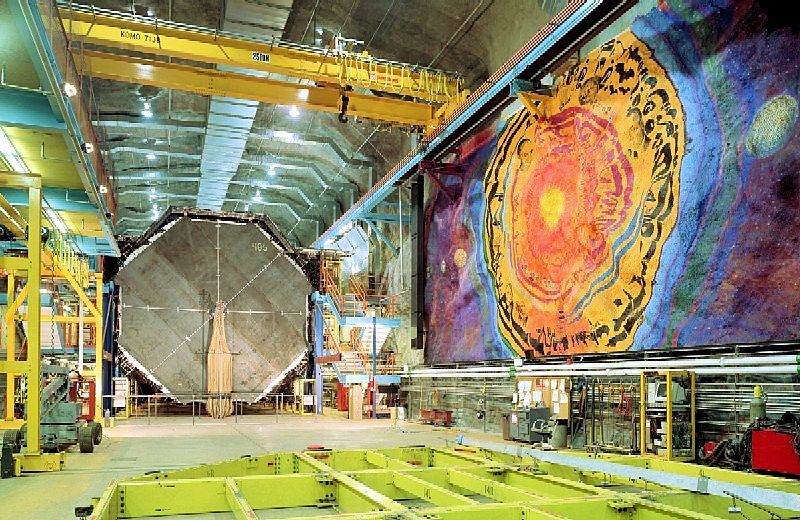
The main tools for such experiments are intense neutrino beams and enormous neutrino detectors. When a supernova was seen to explode in the Large Magellanic Cloud about 170,000 light-years distant, it grew to be easily visible with the naked eye, nearly outshining its galaxy. Even thought most of its energy was emitted in the form of neutrinos, scientists managed to detect just 24 neutrinos out of an estimated 1054 neutrinos.
So what's required of a source of neutrinos for experiments? Most importantly, the source must supply a huge number of neutrinos to the experimental detectors so there is a signal to analyze. The only man made sources known to be suitable are large fission reactors and accelerator-driven neutrino beams.
The current and proposed experiments that appear to have the largest payoff in terms of possible new physics require a large distance between source and detector. For the Fermilab experiments, the detectors are in northern Minnesota, about 740 km (460 mi) distant) from the facility near Chicago.
When brought to full power, the Fermilab neutrino beam will have a current of about a trillion muon neutrinos per second, although these will be delivered as a one millisecond pulse emitted about every second. The angle of divergence of the beam is about 2 arcminutes, so that the beam will only expand to a diameter of about a kilometer at the neutrino detectors.
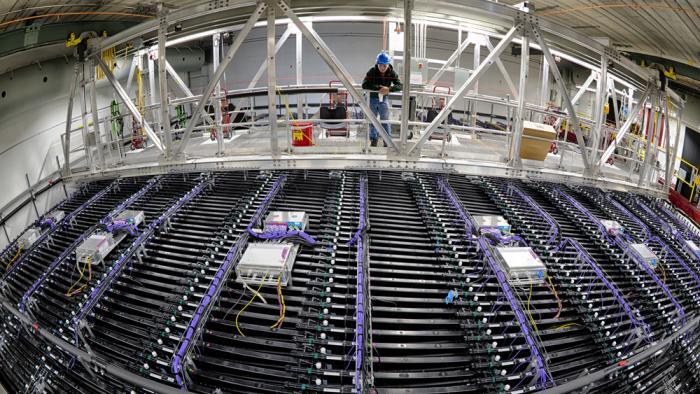
Even with such a powerful beam, the neutrino detectors have to be very large and contain a lot of mass. The MINOS detector has a cross-section of about 60 sq m, and consists largely of 5000 tons of steel, while the NOvA detector, when completed, will have a cross-section of about 250 sq m and an active mass of 14000 tons. In a year's time, these detectors will be struck by about 1016 muon neutrinos per year. Despite this enormous number, only a few thousand counts are expected per year.
Roughly speaking, a neutron beam is formed by accelerating a beam of protons to over 100 GeV of energy. At Fermilab this is accomplished in the Main Injector accelerator, which was used to feed the Tevatron. These protons are dumped from the accelerator ring in a millisecond, and directed onto a graphite target. They wreak havoc on the carbon nuclei, and in the process generate a range of subatomic particles, including in particular pions and antipions. The pions are diverted from the rest of the chaff by properly configured magnetic fields, generated by magnets called "horns", which also focus the pions into a narrow beam. The video following this article explains it all quite clearly.
Why are physicists willing to spend so much of their life's effort (and your tax dollars) to study something as obscure as neutrinos? In short, neutrinos are dead strange – they do not fit naturally into the "basic" Standard Model. As the Standard Model emerges from a collection of well-tested (although not invulnerable) space-time symmetries, maps of neutrino physics bear banners saying "Here there be new physics." Hopefully, Fermilab's revamped neutrino beam will help chart these waters.
Source: Fermilab / Symmetry Magazine