Astronomers have developed a new visible-light adaptive optics (AO) system for the 6.5 meter diameter Magellan-Clay telescope in Chile's Atacama desert. The new AO system replaces the secondary mirror of the telescope with a thin adaptive mirror that can be deformed by its 585 mechanical actuators at a rate of more than 1000 times a second to correct for the image smearing effects of atmospheric turbulence. The result is the sharpest astronomical images ever produced – more than twice as sharp as can be achieved by the Hubble space telescope viewing objects through the vacuum of space.
Space-based telescopes are particularly well suited for cutting-edge astronomy, as they avoid peering through an atmosphere that absorbs and distorts light. Unfortunately, they are also extraordinarily expensive. While the overall cost of the 2.4 meter diameter Hubble runs well into the billions of dollars, a ground-based 2.5 meter telescope costs about $10 million to construct and equip. Even the largest ground-based telescopes currently funded for construction cost about a billion dollars for a 25-30 meter aperture telescope.
At the time the Hubble and other space telescopes were being planned, they offered three critical advantages over ground-based scopes. First, the atmosphere absorbs most of the radiation coming from the cosmos. Only visible light, near-ultraviolet light, some wavelengths of infrared light, and a good deal of the radio spectrum make it through the atmosphere. If you need to observe the cosmos in gamma rays, x-rays, or far-UV light, your telescope needs to be located in space. For infrared observations, light can only partially penetrate the atmosphere in a few narrow bands through millimeter wavelengths, again emphasizing the need for space telescopes. Second, the thermal emission of the atmosphere itself makes the IR sky bright, and still brighter as one looks further into the IR.
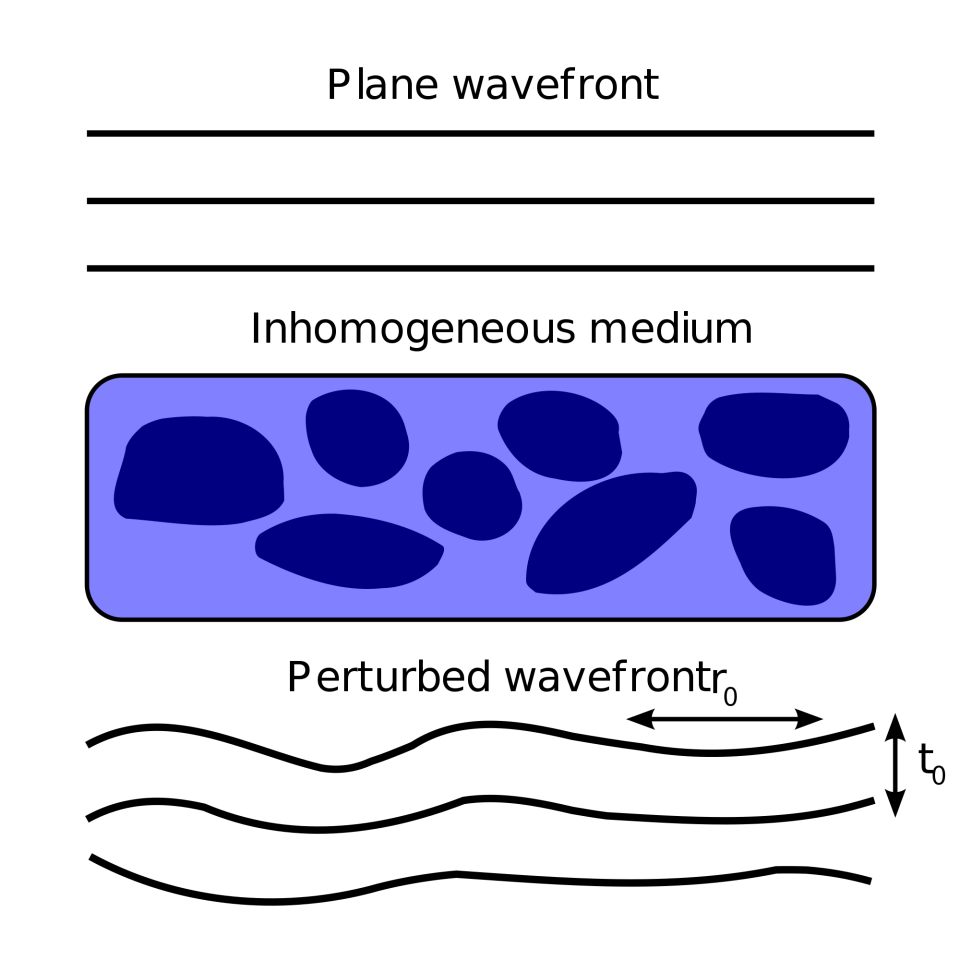
Finally, space telescopes don't have to deal with atmospheric turbulence. Such turbulence distorts the image formed by a telescope, so that ground-based telescopes cannot achieve resolution better than about 0.5 seconds of arc regardless of their aperture. The theoretical resolution of the Hubble is about 50 milliarcseconds (mas), so it can form images about ten times sharper than any ground-based telescope, despite its relatively small aperture.
This situation was changed by the advent of adaptive optical systems in the 1990s. Such systems are able to actively correct the effects of atmospheric turbulence on the image of a celestial object. This is done by using a wavefront sensor to detect changes in the light coming from a point source of light, and then altering the imaging optics to counteract those changes. The point of light in many cases is from a star in the field of view of the telescope, but point sources have also been generated in the upper atmosphere by laser excitation.
Existing adaptive optics systems do not correct wavefront variations well enough to exhibit greatly improved images in visible light, being primarily useful in the infrared. With the advent of the new MagAO system, this barrier has finally been broken, if not shattered.
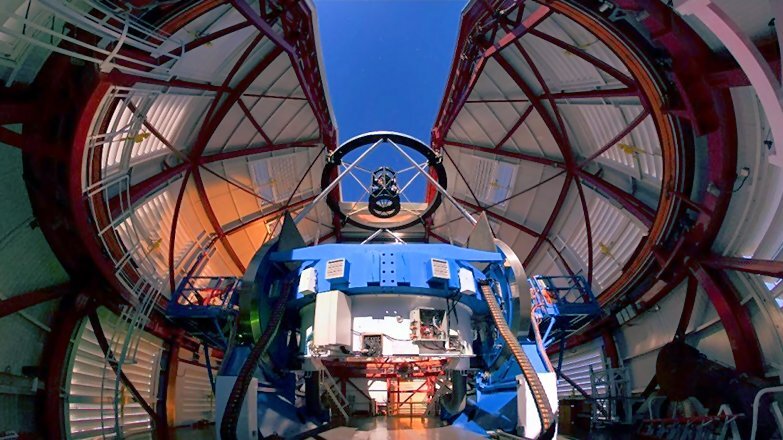
The Clay 6.5 meter telescope has a Nasmyth-Gregorian design, resulting in an f/11 focal plane that emerges through one of the horizontal axes of the telescope mount. The unusual choice of Gregorian optics was made to increase the coupling between the telescope and a specially-design spectrograph.
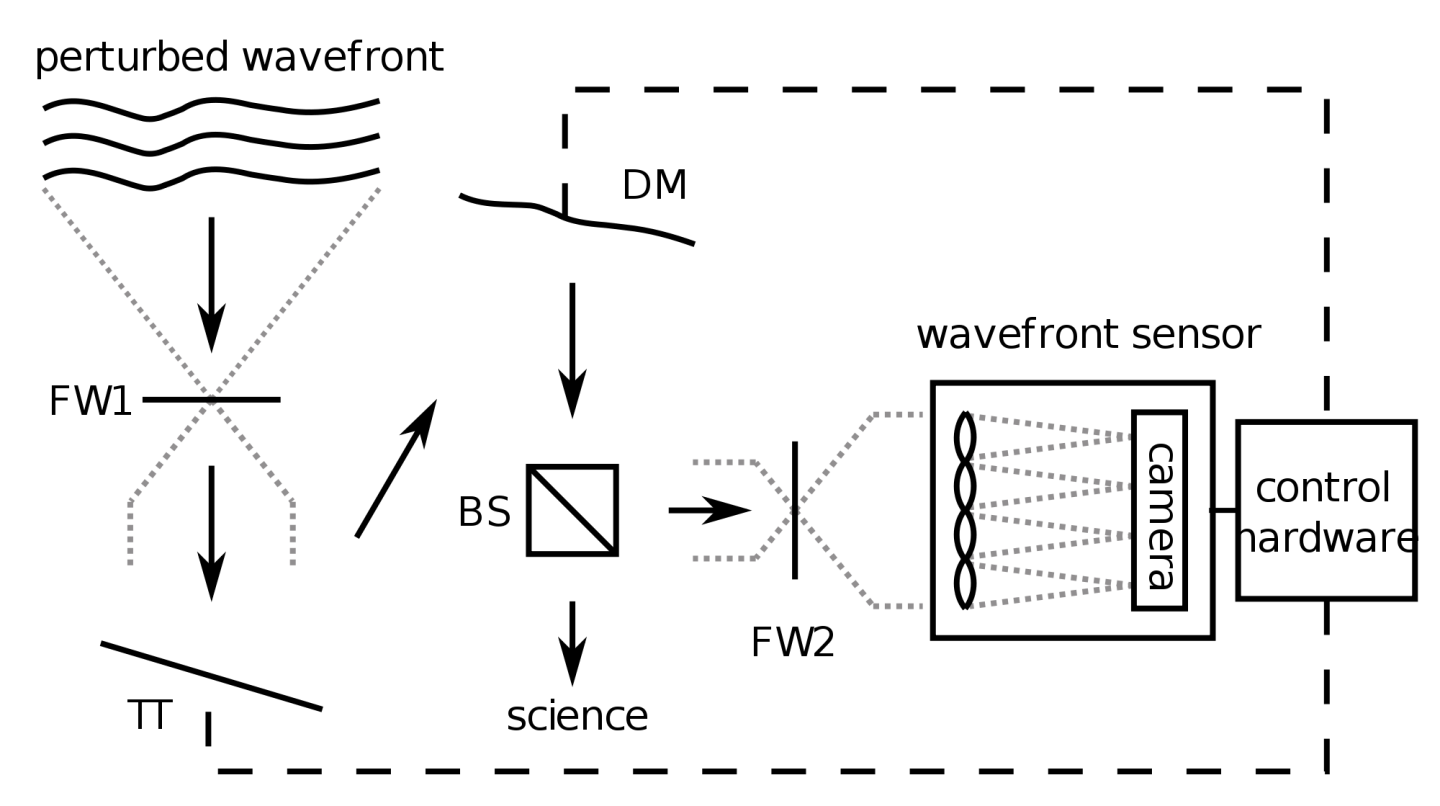
A conventional adaptive optics system would introduce an additional mirror or two into the optical train of the telescope. In the sketch above, these would be the tilt-tip mirror (TT) and the deformable mirror (DM). In operation, the position and shape of these mirrors would be rapidly adjusted to produce an image corrected to an approximation of the clean image (the image in the absence of atmospheric turbulence.)
Jointly developed by the Carnegie Institute for Science, the University of Arizona and Arcetri Observatory in Italy, the 6.5 meter Magellan AO system (MagAO) takes a different approach. It replaces the concave Gregorian secondary mirror of the Clay with an 85 cm adaptive secondary mirror. This mirror, which is held in place by a set of magnetic fields, contains 585 mechanical actuators which adjust its shape roughly each millisecond to remove image distortions.
The wavefront sensor for the MagAO system is a high order pyramid sensor that deconvolutes wavefront errors into 561 modes of distortion, each of which can be compensated by adjusting the shape of the adaptive secondary mirror. The system allows the Clay telescope to resolve details as fine as 0.02 arcseconds (20 mas).
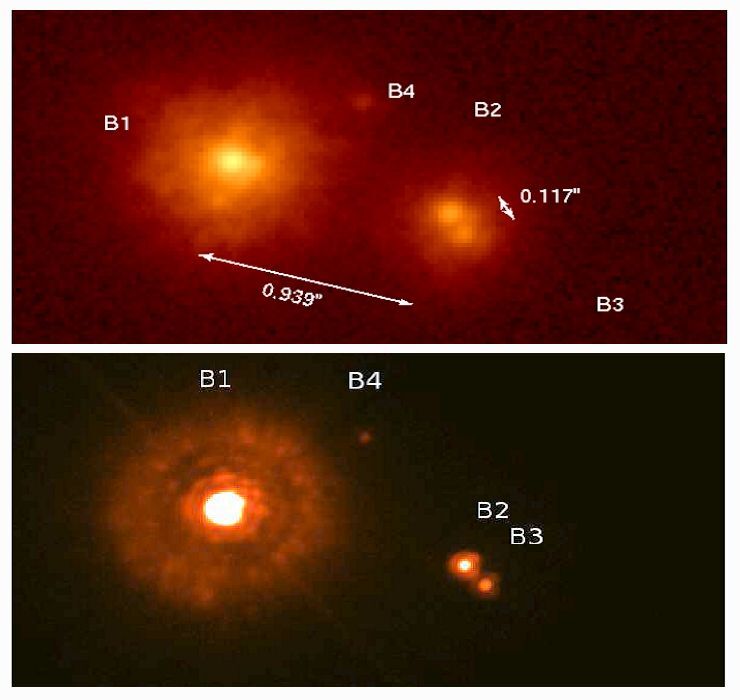
The figure above compares an image of the B star of the Trapezium in the Orion Nebula taken using the 8-meter Gemini telescope using a conventional adaptive optics system with a similar image taken using the 6.5 meter Clay telescope with its new MagAO system. Note the far greater resolution of the image taken by the smaller telescope.
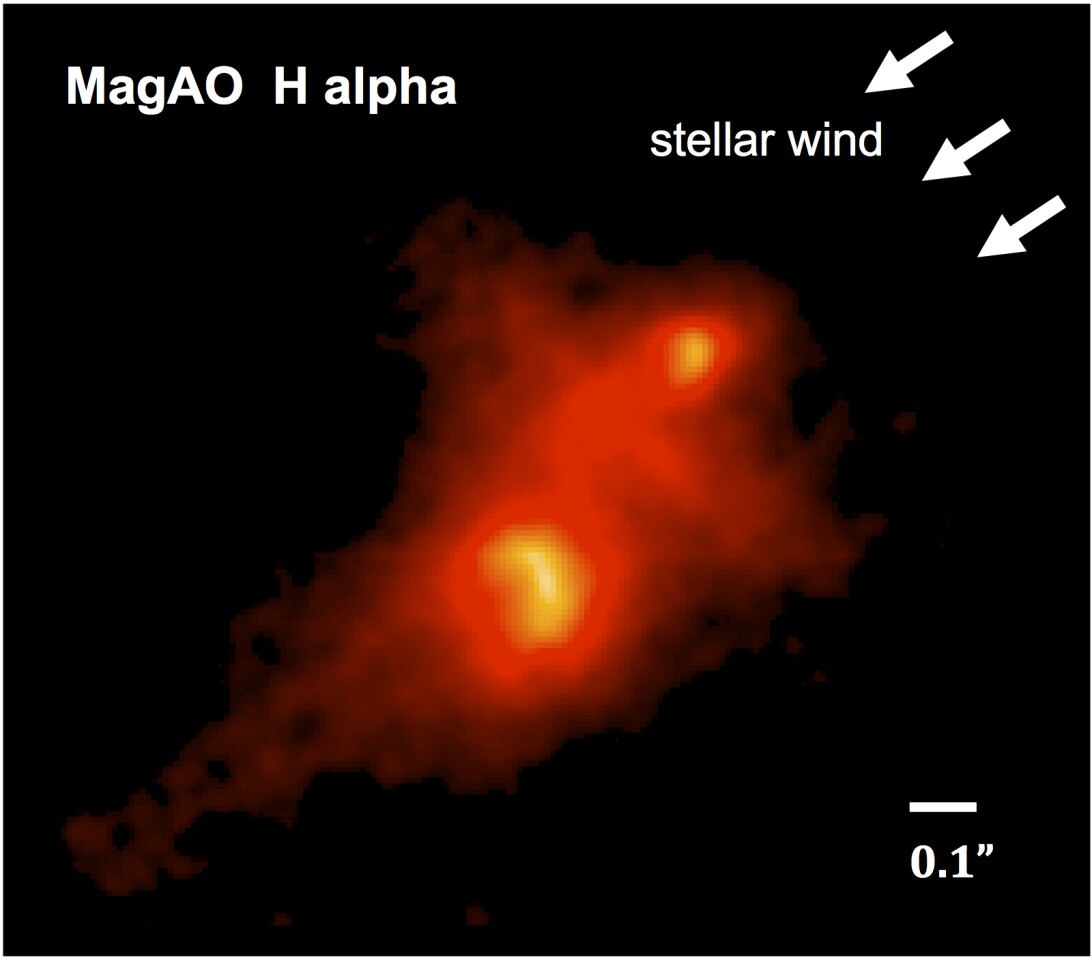
In another remarkable astrophoto, the figure above shows LV1, a very young double star that is sufficiently near the Trapezium that its structure is changed from a pair of stars surrounded by protoplanetary disks to a pair of cosmic dolphins. This is the effect of the intense stellar winds and UV light from the extremely hot O class Theta 1 Orionus C star – the brightest in the Trapezium.
The new MagAO adaptive optical system has proven its ability to produce amazing results on medium-size telescopes in visible light. Further development to enable similar benefits for the use of the largest planned telescopes are expected to follow, opening the prospects of resolving detail on the level of a few mas – about the separation of the Earth and the Moon as seen from Alpha Centauri. Given the rate of telescope refinement and instrumentation evolution, there should be no lack of exciting new observations over the next decade or two.
Source: Carnegie Institution for Science