Researchers have successfully regenerated damaged skull bones in mice by creating a freestanding, biomimetic scaffold that combines a piezoelectric framework and the growth-promoting properties of a naturally occurring mineral. The novel “bone bandage” has wide-ranging potential applications for bone regeneration and regenerative medicine generally.
Piezoelectric materials generate an electric charge in response to applied mechanical stress. Bone is a piezoelectric material. Because it possesses an electrical microenvironment, electrical signals play an important role in the bone repair process, which can effectively promote bone regeneration. However, bone regeneration is a complex process that relies on mechanical, electrical, and biological components.
Current strategies for bone regeneration, such as grafts or scaffolds that release growth factors, have limitations, such as complications at the donor site, limited availability, and high cost. Now, researchers from the Korea Advanced Institute of Science and Technology (KAIST) have developed a pioneering approach to bone regeneration that combines piezoelectricity and a mineral that occurs naturally in bone.
Hydroxyapatite (HAp), a mineral in bones and teeth, plays a role in bone’s structural strength and regeneration. It’s commonly added to toothpaste to remineralize tooth enamel and fortify teeth. Studies have found that HAp enhances osteogenesis (bone formation) and provides a scaffold for new bone growth. It also has piezoelectric properties and a rough surface, making it an ideal candidate for creating scaffolds on which to grow bone.
So, the researchers fabricated a freestanding biomimetic scaffold, integrating HAp within the piezoelectric framework of polyvinylidene fluoride-co-trifluoro ethylene (P(VDF-TrFE)), a polymer film. The independent scaffold, which generates electrical signals when pressure is applied, sets this approach apart from previous research combining HAp and P(VDF-TrFE), which was limited to coatings on metallic prosthetics. The researchers’ novel approach, they say, provides a versatile platform for bone regeneration beyond surface-bound applications.
Comparing scaffolds with and without HAp in vitro showed that cell attachment on HAp scaffolds was 10% to 15% higher. After five days of cell culture, cell proliferation was 20% to 30% higher, and there were approximately 30% to 40% higher levels of osteogenesis on the HAp scaffolds. The findings suggest HAp maximizes the piezoelectric properties of the scaffold and creates an environment similar to the body’s extracellular matrix, the non-cellular component of all tissues that provides essential physical structure and the important cues required for tissue regeneration.
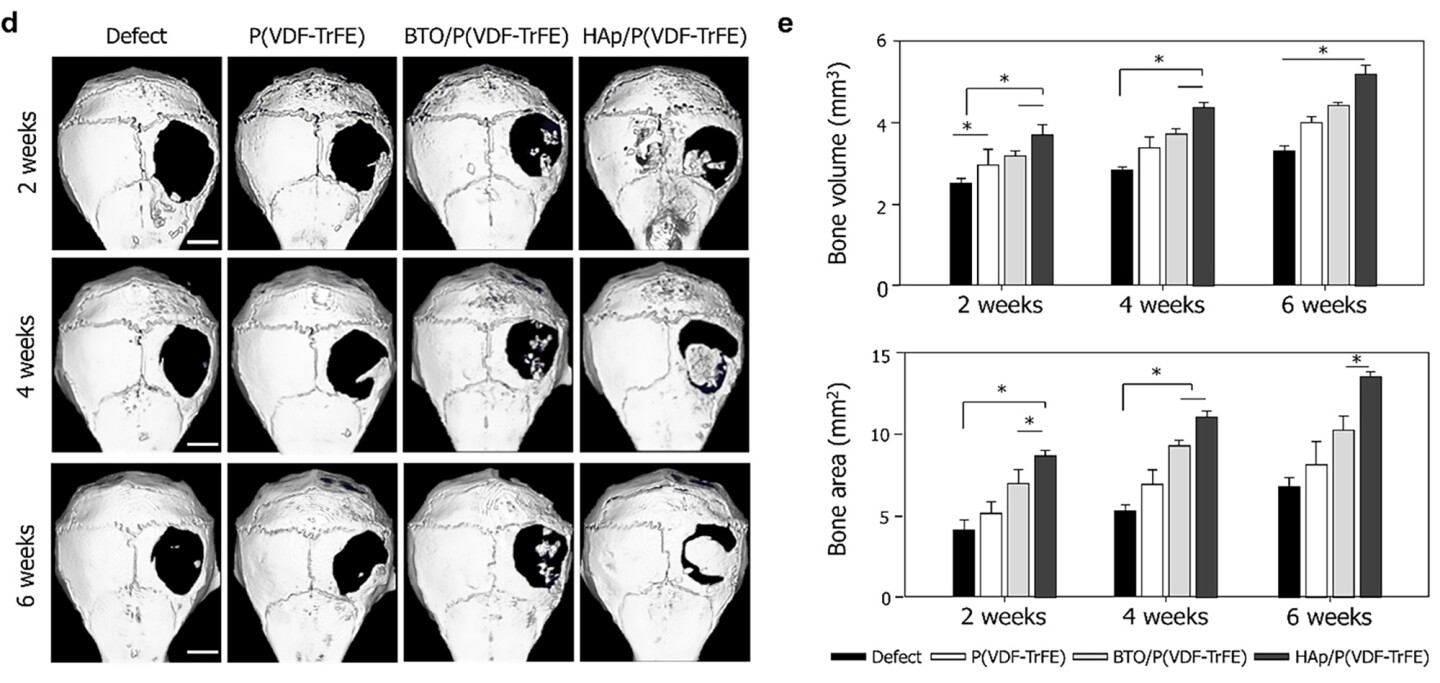
The researchers then tested their HAp/P(VDF-TrFE) scaffolds on mice, placing them over defects in the animals’ skull bones (calvaria). The scaffolds were maintained for six weeks without deformation. All mice survived; no adverse events were observed, including no infection or inflammatory response. After two, four, and six weeks of implantation, bone regeneration was significantly enhanced in the mice with HAp scaffolds fitted compared to no bone formation in the control groups.
“We have developed a HAp-based piezoelectric composite material that can act like a ‘bone bandage’ through its ability to accelerate bone regeneration,” said Seungbum Hong, one of the study’s corresponding authors. “This research not only suggests a new direction for designing biomaterials but is also significant in having explored the effects of piezoelectricity and surface properties on bone regeneration.”
The study was published in the journal ACS Applied Materials & Interfaces.
Source: KAIST