The Large Underground Xenon (LUX) experiment, buried nearly a mile beneath South Dakota, has completed 85 days of seeking dark matter particles. The results are consistent with a null result, and essentially rule out the 8.6 GeV dark matter candidate noted in the data of other experiments.
Back in the 1930s, astronomers began to realize that galaxies in galaxy clusters were moving far too rapidly to be gravitationally bound therein, and concluded that there must be a good deal of "missing mass" they could not see. Similarly, the rate at which stars orbit the center of their galaxy requires additional mass so that the galaxy does not disassemble over cosmic time spans.
As time went on, the evidence mounted that there was some form of matter in the Cosmos which we cannot directly see. Indeed, recent cosmological models based on a wide range of independent evidence suggest that there is about five times more so-called dark matter in the Universe than there is ordinary matter.
The evidence associated with the cosmic microwave background is particularly significant, in part because the data contained in the CMB has remained largely untouched since about 379,000 years following the Big Bang, when the Universe became transparent. Not only does the information encoded in angular fluctuations agree with the existence of massive amounts of dark matter, but in (essentially) the entire lifetime of the Universe, the CMB has accumulated no sign of interaction with the dark matter, leading to the idea that dark matter must not interact through electromagnetic or nuclear binding forces, but only through the weak force and gravity.
Despite having a good deal of inferential evidence for the existence of dark matter, efforts to detect a specific particle or influence responsible for this massive amount of matter have been fruitless to date. Many suggestions exist, such as a distribution of relatively small amounts of cold matter (MACHOS - Massive Compact Halo Objects), Weakly Interacting Massive Particles, or WIMPs, axions (suggested in order to plug a hole in the Standard Model of particle physics), and a host of others. Other suggestions, such as altering the theory of gravity to force agreement with observations without requiring any additional matter, so far fail to agree with enough of the relevant evidence for dark matter to be taken seriously.
At this point, cosmology and particle physics are in a quandary about dark matter, which explains why it is receiving so much attention by researchers. People tend to hop on any bit of statistically insignificant data that might point more or less toward the true resolution of this puzzle. For example, over the past two years two groups of observers have found some evidence that there exists a wholly unexpected candidate particle for dark matter with a mass of 8.6 GeV, or about nine times the mass of a proton. The mass is far below where WIMPs are expected to form, but science, if it is about anything, is about surprises.
LUX is presently the most sensitive recoil-based dark matter detector on the planet, and has just finished roughly three months of observations aimed at detecting the collision of particles of dark matter with xenon atoms. Such measurements are relatively independent of just what a dark matter particle is. If it interacts predominantly via the weak force, it could be detected by LUX.
The detector is located 1,480 m (4,850 ft) below ground at the Sanford Underground Research Facility in Lead, South Dakota. A 7.6-m (25-ft) diameter by 6.1-m (20-ft) tall cylindrical water tank containing ultrapurified water provides shielding to the detector from the radioactive elements present in the walls of the mile-deep canyon. The cosmic-ray background is already reduced by a factor of nearly a billion by the rock overhead.
The LUX detector holds 370 kg (816 lb) of liquid xenon, about 118 kg (260 lb) of which is active as the primary LUX scintillator (an atom that gives off light when struck by another particle). The remainder of the liquid Xenon acts to provide additional shielding for the active portion of the experiment, and to separate that portion from direct contact with the structural materials of the detector, which are ever so slightly radioactive.
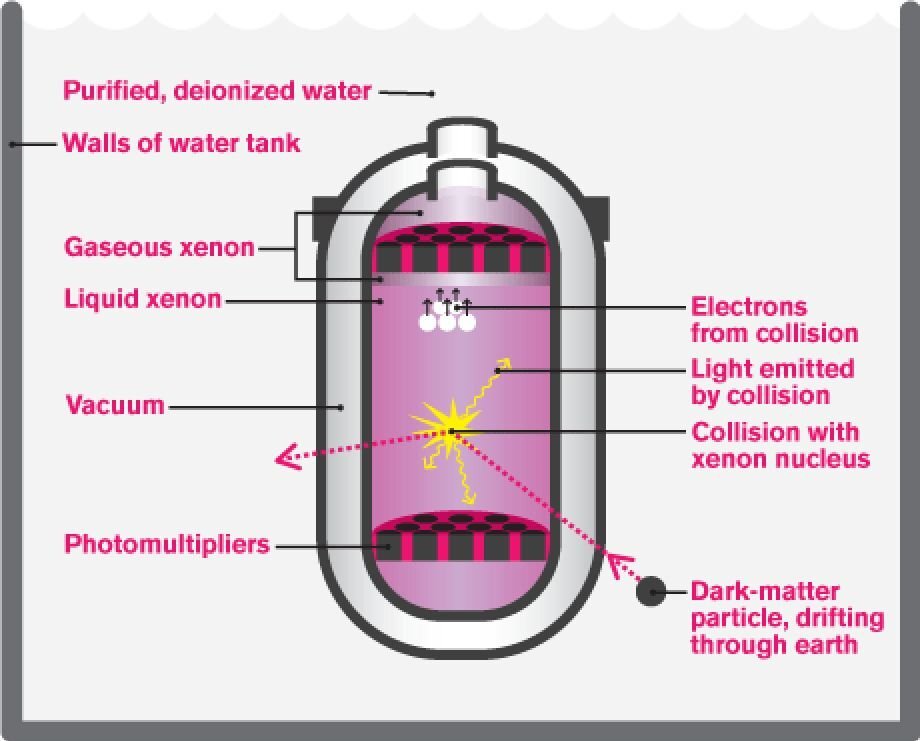
The active detector is a dual-phase time-projection chamber measuring 47 cm (18.5 in) in diameter and 48 cm (19 in) in height. When incident particles (including dark matter particles, if any) collide with xenon atoms in the chamber, the xenon atoms recoil and produce a prompt flash of light, which the lUX team call the S1 event.
Ionization of the xenon near the collision also occurs, if the collision is energetic enough. There is an electric field of 181 V/cm applied within the xenon liquid. The electrons freed by the ionization drift upwards under the influence of this field, and cause a second pulse of light (S2) when they pass into the gas above the liquid xenon. The time (S2-S1) it takes the electrons to drift to the surface tells how deep the S1 event was. The horizontal location of the S1 event is determined by analyzing the signals from the bottom array of 61 photomultiplier tubes.
The background counting rate of the detector in place underground was carefully studied. About 1,000 background events could be expected during the 85 live days of data collection, the vast majority of which would not show the proper signature to be confused with a recoil event.
Some 84 million events triggered the detector during the 85 live days of operation. Once multiple scattering events (recognized by multiple S1 and S2 events very closely spaced in time) were eliminated from consideration, 6.6 million remained. When events that did not have the signature of true recoil events occurring within the central core of the time-projection detector were also eliminated, only 160 events remained, a rate of about two per day.
The number of events are sufficient immediately to rule out the 8.6 GeV WIMP candidate possibly seen in the Berkeley CDMS II data. Given the collision cross-section of 2 x 10^-41 cm2 (derived from the data), LUX should have seen about 1,550 events attributable to dark matter during this data run. Instead the researchers saw none, which contradicts the CDMS II observations so dramatically that one of the experiments must be in error. As the LUX run comprised nearly 100 times more data, CDMS II is probably in error.
To extract possible dark matter collision events from an unknown residual from background radioactivity required complex statistical analysis of the 160 possible events. To make a long story short, the spectrum of possible dark matter events is consistent with no actual dark matter events being detected – in other words, a null result.
This question can be flipped on its head, to ask if a dark matter event could successfully hide from statistical analysis. When this was tried by the LUX team, it found that between 2.4 and 5.3 (depending on dark matter mass) dark matter events could hide in the data, but the error bars make these numbers indistinguishable from zero.
The bottom line is that this early LUX data run found no evidence for the existence of dark matter, and was able to tighten the bounds on the collision cross-section of dark matter and normal matter by a factor of at least three. The LUX team is now looking at longer data runs, but for the moment the best data we have does not disclose any form of weakly interacting dark matter. What the LUX experiment has accomplished is to narrow the field of acceptable candidate dark matter particles, a process that will hopefully continue until this puzzle is solved.
Source: LUX Experiment