It sounds like sci-fi: normal matter has an “evil twin” that annihilates as soon as the two come into contact. But this antimatter is very real, and despite decades of study it remains very mysterious. So what actually is antimatter? Where is it? Why is it important that we understand it? And why hasn’t it already destroyed the universe?What is antimatter?
As strange as it sounds, antimatter is essentially just like regular matter, except its particles have the opposite charge. But that simple difference has some major implications – if ever a particle and its antiparticle should meet, they will annihilate each other in a burst of energy.
Lucky for us, antimatter is extremely rare. It’s produced naturally in tiny amounts in cosmic ray interactions, during hurricanes and thunderstorms, and as part of some types of radioactive decay – in fact, anything with potassium-40 in it will spit out the occasional antimatter particle. That includes bananas and yes, even you. But don’t worry, it won’t get far before it collides with an electron and vanishes again.
Artificially, antimatter is mostly produced in particle accelerators like CERN’s Large Hadron Collider, but again only in minuscule amounts, and it usually doesn’t last long.
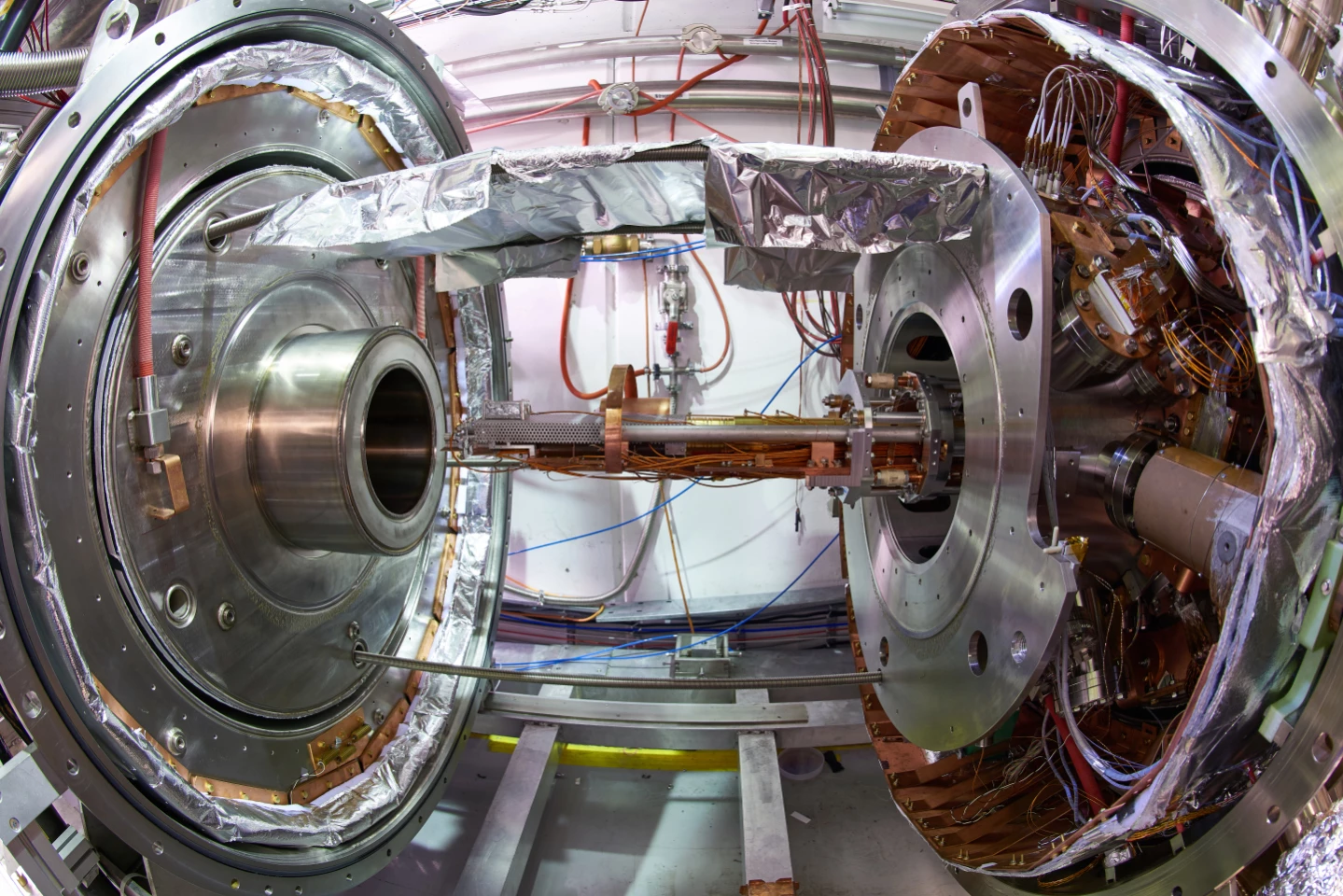
Every particle has its equivalent antiparticle – for example, there’s the antiproton, the antineutron, and the antielectron (better known as the positron). Some particles, such as photons, are actually their own antiparticles.
These antiparticles can also link up to form antiatoms, so for example an antiproton and an antielectron can form an antihydrogen atom. Every element should have an antimatter equivalent, and they should have all the same properties as their regular matter counterparts except for charge.
Of course antimatter is not inherently “worse” than normal matter – we just define it as “anti” because it’s the inverse to the stuff we’re used to. But if somewhere out there, there are creatures made of antimatter living on an antimatter planet, orbiting an antimatter star in an antimatter galaxy (which, by the way, is all theoretically possible) – they’d probably switch the matter and antimatter labels around.
It might be easy to confuse antimatter with dark matter, but the two are very different. Dark matter is hypothesized to be spread out all through the universe, and while there’s plenty of evidence that it exists, it still evades direct detection. Antimatter, on the other hand, is extremely rare but has been experimentally confirmed and is the subject of constant study.
So how are we studying antimatter?
Scientists at facilities like CERN can create antimatter by smashing certain particles together in an accelerator, which produces showers of matter and antimatter pairs. When these pairs are separated, the antimatter can be saved and studied.
However, it’s a difficult procedure, and as such only a few dozen nanograms have ever been produced artificially. This also makes it far and away the most expensive material in the world to make, with scientists estimating that it costs up to US$25 billion per gram. Part of the difficulty and cost comes from storage, because of course it’s not as easy as just sticking it in a jar, since it will annihilate most containers on contact.
So, scientists use what’s called a Penning trap. Antimatter particles are suspended inside a vacuum chamber by electromagnetic fields, which keeps them away from the sides. Using this method, scientists first managed to trap atoms of antihydrogen for a few fractions of a second in 2010, then extended that to over 16 minutes in 2011. The current record is storing antiprotons for 405 days.
If storing antimatter seems tricky, transporting it is a whole other level of challenge. In 2020, CERN detailed a new design for a trap that could be used to move large quantities of antimatter over longer distances.
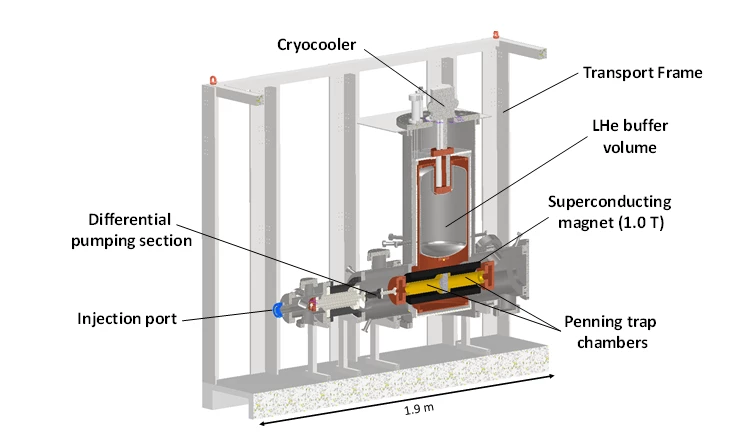
The device, called BASE-STEP, would be made up of two Penning traps, one that receives and releases antiprotons and the other to store them for transport. The traps would be surrounded by a 1-Tesla superconducting magnet to steady them, while a layer of liquid helium keeps the system cool for long periods of time. The whole unit is small enough to load onto a truck, which could then transport the antimatter to other facilities for more detailed study.
It’s enough to make you wonder why we bother at all, but antimatter has great technological potential. In fact, it’s already found one useful application which you may have experienced yourself.
What could we use antimatter for?
If you’ve ever had a PET scan, you’ve had doctors watching your body for antimatter annihilation events inside you. It’s right there in the name – PET stands for Positron Emission Tomography, and a positron is the antimatter version of an electron.
PET scans work by injecting patients with a radioactive tracer chemical, which emits positrons as its particles decay. These positrons will then collide with an electron in a patient’s tissue, giving off gamma ray photons which are captured by a specialized camera. By tracing these events doctors can then reconstruct 3D images of organs and tumors.
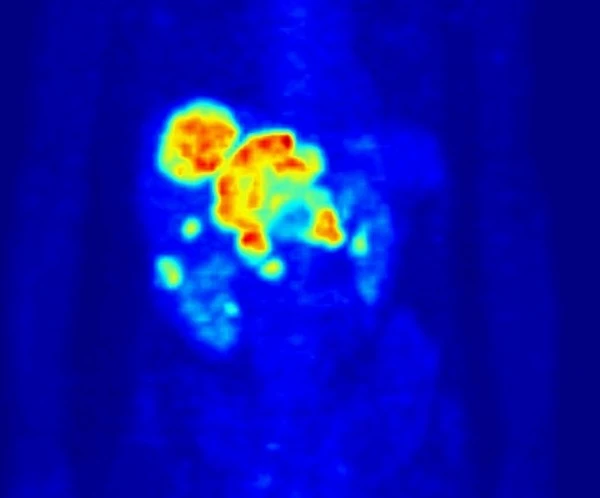
If antimatter can ever be produced or collected at large scales, we might be able to use it for more revolutionary applications. The energy released when matter and antimatter collide is enormous – just one gram of each would produce about the energy of a 40 kiloton atomic bomb. Harnessing that could help propel spacecraft of the distant future through the universe very efficiently – just a few dozen milligrams of antimatter would be enough to send a ship to Mars.
The darker side, however, is that antimatter could make a weapon of untold destructive power, but thankfully, the prohibitively high cost keeps that in the realm of Dan Brown novels. For now.
But before we make any grand plans for antimatter, we need to study it in far more detail. After all, there are some fundamental questions we still don’t have the answers to.
The big antiquestions
Besides having the opposite charge, matter and antimatter should be basically the same, and follow the same laws of physics – but the emphasis there is on “should.” Assumptions don’t make for solid science, so physicists have been double-checking the basics just in case, because any anomalies could hint at a whole new chapter of the Standard Model of particle physics.
For example: every element and compound has a unique fingerprint called its emission spectrum, based on which wavelengths of light they absorb and which they emit. According to the Standard Model, matter and antimatter atoms of the same element should have the same spectrum, but it wasn’t until 2016 that CERN scientists finally checked. The team zapped atoms of antihydrogen with a laser to measure its spectrum, and found that it matched that of regular hydrogen.
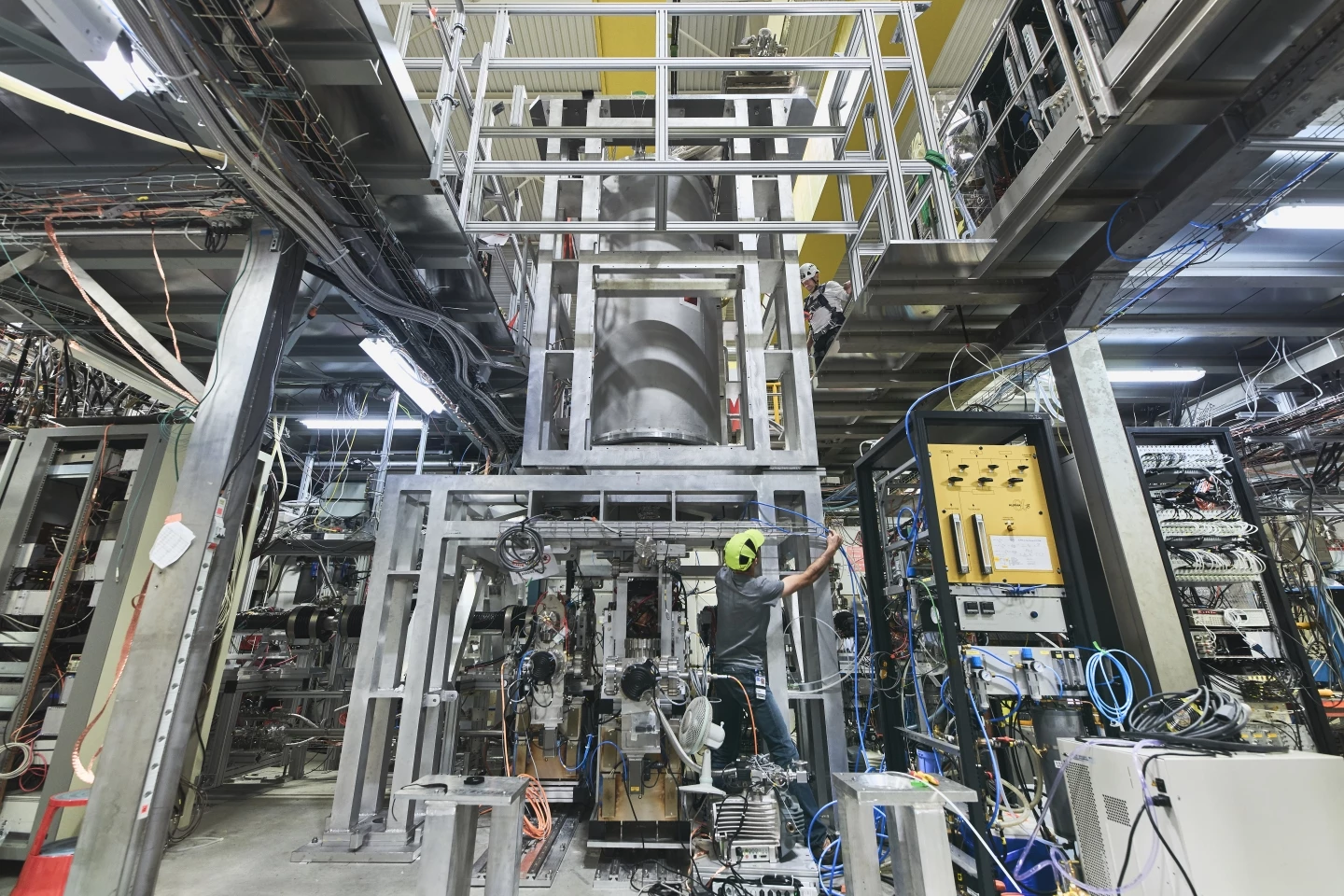
Another important question is whether antimatter reacts to gravity the same way regular matter does. Again, it’s expected that it should, but there’s about a one in a million chance that antimatter might actually fall upwards instead. It sounds like a pretty basic thing we should already know, but all work with antimatter so far has had to suspend it in electromagnetic traps.
CERN has two separate experiments in the works to test the idea, known as GBAR and ALPHA-g. In both cases, the experiment design is pretty simple – switch off that trap and see if the annihilations take place below or above it. Hopefully we don’t have much longer to wait for the results.
But there’s another major cosmic mystery that we could solve by studying antimatter – why are we even here at all?
Matter-antimatter asymmetry
According to the Standard Model of particle physics, the Big Bang should have produced matter and antimatter in equal amounts. But if so, the entire contents of the cosmos would have annihilated itself through collisions over time, leaving the universe a very empty place today.
Obviously, that didn’t happen. So what happened to all the antimatter?
It might be out there somewhere. Theoretically, antimatter should be able to clump together into stars, planets and galaxies just like regular matter (as long as there’s no regular matter around to annihilate it). That means there could be corners of the universe where antimatter dominates.
And it may not be as far away as you might think. Some scientists suggest that antimatter stars could be lurking in our own galaxy, shining just like regular stars. However, we might be able to identify these “anti-stars” through the unusual gamma ray bursts they’d give off as specks of matter touch them.
But this might also unravel the idea. Even if matter and antimatter regions of the universe were separated by vast stretches of intergalactic space, there would still be fairly regular annihilation events taking place along the boundaries. This would result in clear gamma ray signals, which have not been observed, making it unlikely there are antimatter-dominated regions in the observable universe.
Instead, the main line of reasoning goes that in the early days of the universe, something caused an imbalance in the matter-antimatter ratio, so that there ended up being a tiny bit more of one than the other. That would mean that all the matter in the universe today is just a minuscule fraction – as little as one 10-billionth – of what once existed, leftover after a cosmic cataclysm of antimatter annihilation.
So what could have caused that imbalance? There are a few hypotheses.
One idea says that we have neutrinos to thank for our existence. These neutral subatomic particles are their own antiparticles, and it’s thought that they could have converted some antimatter into matter as the early universe underwent a phase transition.
Other subatomic particles like the charm meson have been caught switching between matter and antimatter in the lab. If for some reason the switch from antimatter into matter is easier for them than the opposite, they could have created the imbalance early on in the universe's history.
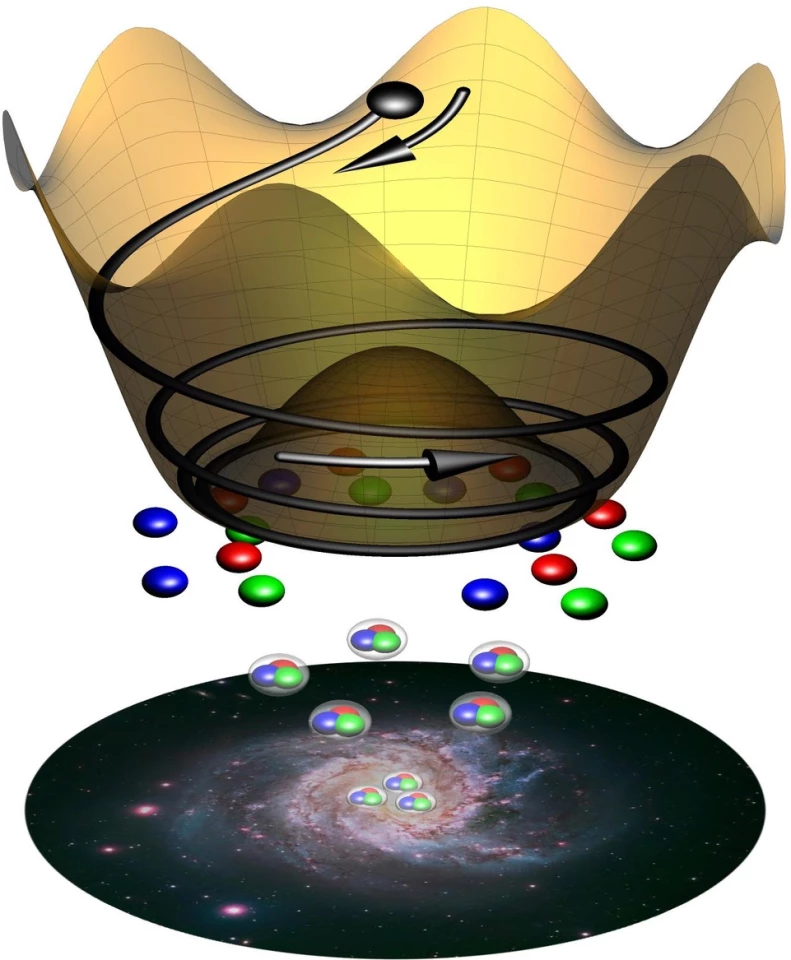
Another story points to a hypothetical particle called an axion. These are predicted to be incredibly light, have no electric charge, and float around the universe in waves rarely interacting with other matter. According to one study, the axion field began to oscillate in the early universe, creating a tiny fraction more matter than antimatter.
Intriguingly, axions may turn out to be a very convenient particle. Their existence could solve not just the matter-antimatter asymmetry problem, but two other cosmic conundrums at the same time – they’re also a dark matter candidate, and they plug up another hole in our understanding of particle physics, known as the Strong CP problem, too.
The inherent enigma of antimatter will likely continue to perplex scientists for a long time to come. Lab experiments and astronomical observations will no doubt provide new clues that could help us understand the evolution of the universe, the limits of the Standard Model of particle physics, and how to harness this strange substance for uses we can’t even imagine.
Check out our other physics explainers on dark matter and dark energy.