In 2012, scientists at the National Physical Laboratory (NPL) and the University of Leicester came up with an idea to replace radio-based space navigation with a cosmic GPS that uses pulsars as a sort of deep-space lighthouse. Four years later, they've published a paper as part of a ESA feasibility study to see if the idea is practical and shown how it could be used to fix a spaceship within a radius of two kilometers (1.2 mi) at the distance from Earth to Neptune.
Today's deep space missions use navigation based on Earthbound stations, such as NASA's Deep Space Network (DSN) or ESA's European Space Tracking (ESTRACK) network. These systems use radio signals traveling between Earth and the spacecraft to fix its position and plot its trajectory at distances well past the orbit of Pluto, but it's a system that is showing signs of obsolescence and limitation.
One problem is that relying on a handful of ground stations isn't a very secure way of navigating. It's a bit like going on a car trip with one road map and a dog who might decide to eat it. Another factor is that the deep space networks can only handle so many missions at any one time, and the past couple of decades have seen a major increase in traffic beyond lunar orbit. Worse, some of these probes are now so far from Earth that it takes nearly a day for a signal to reach a spacecraft and return.
One solution is to come up with a navigation system that isn't so dependent on the Earth. Since it isn't feasible to set up an outer space Trinity House to strew the cosmos with lighthouses and navigation buoys, ESA looked to landmarks (spacemarks?) that are already there.
In this case, it's pulsars. These are dead stars that have burned up all their hydrogen and helium fuel and have collapsed in on themselves. They are so massive that the pressure formed by the collapse is even stronger than the forces that keep atoms in shape. The electrons and the protons in the star's atoms were squeezed together to form neutrons, and the star is now made up of a new material called "neutronium," which is essentially one big mass of neutrons.
These neutron stars are called pulsars because as they collapse the law of the conservation of angular momentum makes them spin at incredible speeds of thousands of revolutions of minute, and as they spin they spew out intense beams of radio energy and X-rays.
The result is a star that flashes in a bright pattern that is so regular that when the first pulsar was discovered in the 1960s, it was called LGM-1 or Little Green Men One because the scientists thought that it might be a signal from another intelligent race.
Though that didn't turn out to be the case, these pulsars were soon seen as a means of navigation. In 1972 NASA put together the famous plaque for the Pioneer 10 and 11 space probes, which became the first manmade objects set on an interstellar trajectory. Among other information, the inscription included a map that used pulsars to show the position of our Solar System.
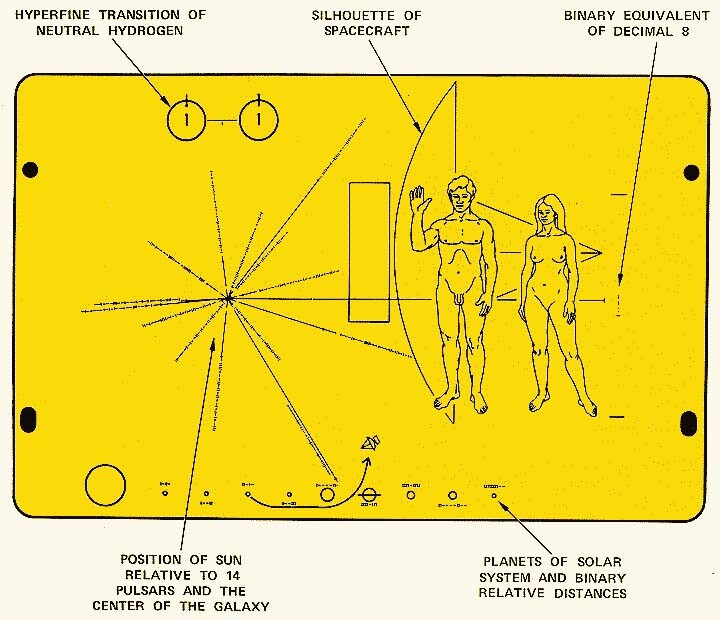
The Pioneer plaque was a novel idea, but just pointing to a pulsar in the sky isn't a very accurate form of navigation. It's fine for locating the Solar System, but for pinpointing, for example, the Earth, it would have been a case of "Go to Jupiter and then ask." What was needed was something more accurate.
The ESA pulsar system, called XNAV, is different from a quasar-based navigation system also being developed by ESA that uses long-baseline radio navigation for spacecraft, with quasars thrown in to compensate for atmosphere-induced errors. XNAV is more like traditional sea navigation in that it's "barycentric," which is an impressive word for taking your bearings.
In principle, XNAV is very simple. A navigator on a ship, among other methods, finds the way by using a compass. The needle pointing North provides a baseline and by taking bearings on known landmarks, such lighthouses, buoys, and mountains, lines can be drawn on a chart showing their directions. Where these lines intersect form a (hopefully) tiny triangle and the center of that triangle is the ship's most probable position.
XNAV does the same thing, but in three dimensions instead of two and with a few complications thrown in. First, it uses the Sun or the Earth as a baseline, and an X-ray telescope, because pulsars aren't visible and X-rays are more accurate for taking fixes than the alternative radio waves. Also, X-ray telescopes are much smaller, lighter, and less expensive than radio telescopes.
According to NPL, the X-ray telescopes don't even need to be as large or expensive as the ones currently used in space because they only need to do one task instead of many. For XNAV, these are full-image telescopes because by taking a complete X-ray image of the pulsar, cosmic "noise" can be minimized and the fix made more precise and accurate.
In theory, XNAV works the same as our sailor. It identifies one or more pulsars, takes a fix, and calculates the spacecraft's position. However, the XNAV team has found that there are a few snags that need to be overcome, so they ran a series of simulations run to see how accurate such a pulsar-based navigation system would be and what sort of instruments would be needed to make it practical.
One thing they found was that not just any pulsar will do. Pulsars tend to be very young objects. One of the best known, which sits at the heart of the Crab Nebula, is only a thousand years old. Because they're so young, pulsars tend to be unstable and their characteristics can change without notice on a daily basis. So, out of the 2,000 known pulsars, the XNAV team trimmed the list down to a handful of "millisecond pulsars," which are a small, relatively stable set of relatively old pulsars.
The other problem with pulsars is that though the XNAV team likes to compare them to GPS satellites, they're obviously not GPS satellites. For GPS, the engineers program the satellites that circle the Earth to broadcast a signal for GPS receivers to lock onto, much like XNAV and the pulsars. However, the GPS signal includes an identification of the satellite, a time signal, and other information. The pulsar just blasts out what nature gave it. Something needs to be added.
First, the XNAV system on the spacecraft would carry the most recent information on the pulsars. Since even a few days could introduce errors, these would be minimized by taking readings on a single pulsar over a period of hours, or on several pulsars – like looking at more than one lighthouse.
Second, the spacecraft would keep in touch with the deep space tracking networks, which would act as a baseline and make sure the pulsar data is updated and the XNAV system's clock is properly synchronized. The later is particularly important because a super-accurate clock would allow XNAV to take a doppler reading on the incoming pulsar signal and calculate the spacecraft's velocity.
An even better solution is for the spacecraft to carry an atomic clock aboard. This would allow the XNAV to operate for months or years without contact with Earth because there would be no need to synchronize the clocks.
For their feasibility study, the XNAV team ran a series of simulations taking into account variables such as how accurate and up to date information about the quasars is, how far the spacecraft is from Earth, and how many pulsars are involved. What they found was that by taking a ten-hour observation of a single pulsar, XNAV could take a fix on a spacecraft that is accurate to within two kilometers (1.2 mi) at a distance of 30 AU (3 billion mi, 4.5 billion km), which is the distance from Earth to Neptune.
In another scenario, a simulated manned mission to Mars, out of touch with Earth for three months, took observations on three pulsars and made a position fix with an accuracy of 30 km (19 mi).
"Up until now, the concept of pulsar-based navigation has been seen just as that - a concept," says John Pye, Space Research Centre Manager, University of Leicester. "This simulation uses technology in the real world and proves its capabilities for this task. Our X-ray telescope can be feasibly launched into space due to its low weight and small size; indeed, it will be part of a mission to Mercury in 2018. NPL's timing analysis capability has been developed over many years due to its long heritage in atomic clocks. We are entering a new era of space exploration as we delve deeper into our solar system, and this paper lays the foundations for a potential new technology that will get us there."
The team's results were published in Experimental Astronomy.
Source: National Physical Laboratory