With the world’s first laser enrichment plant having received a construction and operating license from the US Nuclear Regulatory Commission in 2012, the stage has been set for a radical change in the industry. So how does laser enrichment work, and what commercial benefits, along with proliferation concerns, does this new process present compared to current methods?
Nuclear power plants generally benefit from uranium enrichment. By increasing the abundance of U-235 in uranium fuel rods from the natural abundance of 0.71 percent to 3 percent or greater, fewer compromises need be made to reach gigawatt power levels. A few reactors use uranium enriched to about 20 percent U-235, and at the extreme, some nuclear submarines use fuel enriched to about 93 percent.
Nuclear weapon designs based on uranium fission always benefit from uranium enrichment. Few proliferation concerns arose when the expensive and technically demanding method of gaseous diffusion was the only practical approach to enrichment, as only nation-states with enormous resources were likely to be able to use that process to obtain weapons-grade fuel. Given centrifuge and now laser-based enrichment technologies, this is no longer the case.
Three general types of uranium enrichment have been developed into large-scale processes: gaseous diffusion, centrifuge cascades and now laser-based enrichment. Of these, gaseous diffusion facilities have been considered to be too large, expensive, and technically demanding to represent a significant proliferation hazard. Such facilities are now obsolete and currently the dominant technology for uranium enrichment is the high-speed centrifuge cascade. Now that laser-based enrichment methods have arrived, will highly enriched uranium become significantly more accessible to rogue nations and terrorist groups? Let's take a look at this new approach in comparison to centrifuges in an effort to answer this question.
Centrifuges
A gas centrifuge is a hollow cylindrical tube that is rapidly rotating around its long axis. The rotation produces a centrifugal force on a uranium hexafluoride gas in the cylinder, which acts to force the gas toward the wall of the centrifuge. This force is stronger on uranium hexafluoride containing U-238 than on the same material that contains U-235. As a result, the gas near the centrifuge wall is enriched in U-238, while the gas near the axis of rotation is enriched in U-235. The largest centrifuges used in uranium enrichment hold only about 15 grams of uranium, so an enrichment plant must include many parallel paths to produce commercially viable quantities of enriched uranium.
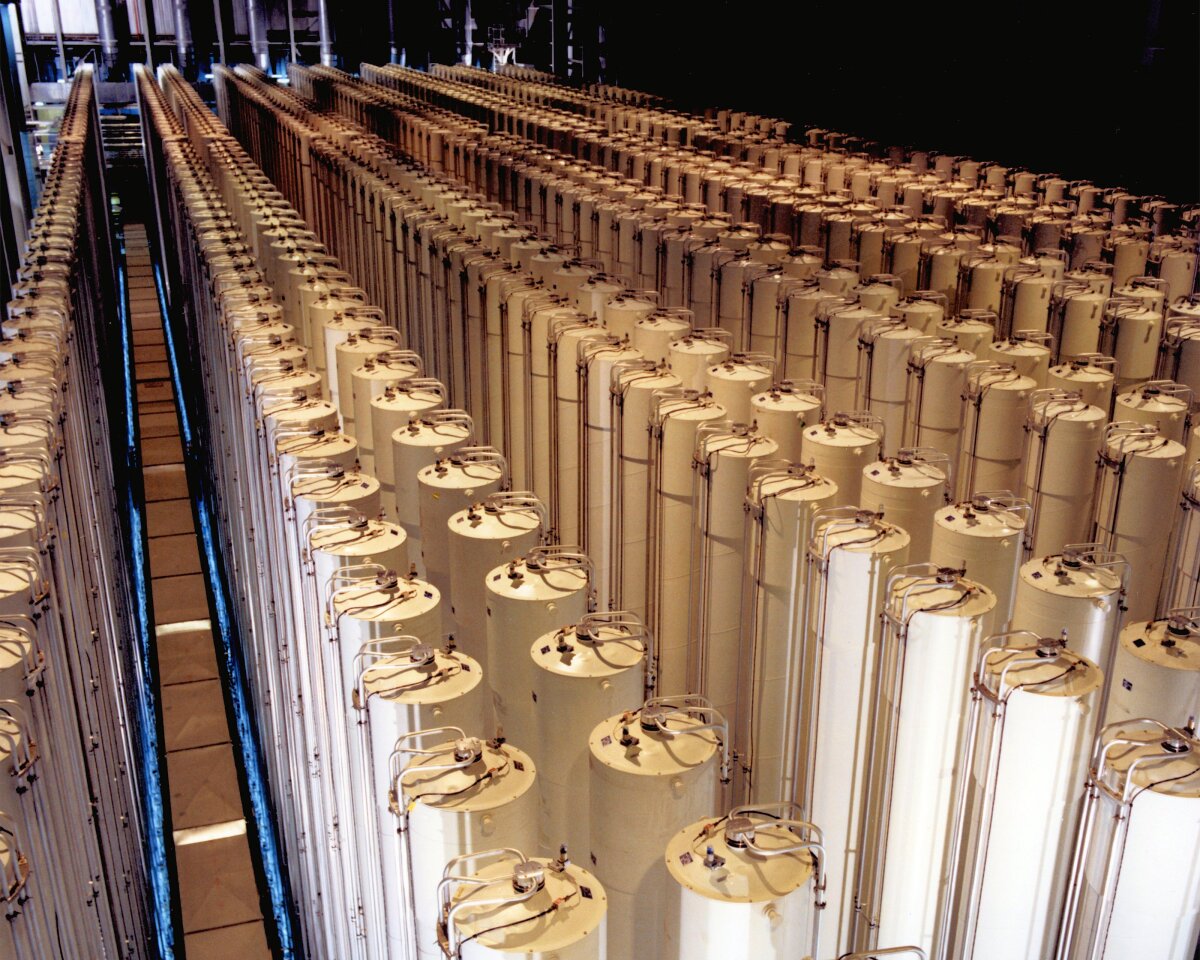
Typically, an enrichment cascade would require about seven stages to produce uranium enriched to five percent U-235, and about 20 stages to produce weapons-grade U-235. Centrifuge cascades have been the main route toward nuclear proliferation, most notably in Pakistan, Iraq, Iran, Libya, and North Korea.
Laser isotope separation
The new player in the game is enrichment techniques that depend on laser excitation to separate isotopes. The basis for all laser-based enrichment schemes is that the amount of energy required to put a uranium hexafluoride molecule containing an atom of U-235 into an excited state is slightly smaller (by about 0.1 percent) than the energy required when the uranium atom is U-238. It turns out that carefully tuned photons from a 16 micron laser will excite the U-235 containing molecules, but not the U-238 containing molecules. As only molecules containing U-235 are in an excited state, this gives a handle with which to differentiate, and eventually to separate, the two isotopes.
To be built by GE and Hitachi, the first commercial-scale U-235 laser enrichment facility licensed for production will use an Australian-developed laser enrichment technology known as Separation of Isotopes by Laser Excitation (SILEX). Currently Silex has completed its phase I test loop program at GE-Hitachi Global Laser Enrichment’s (GLE) facility in North Carolina. When the commercial plant is built, its target enrichment level will be 8 percent, which puts it on the upper end of low-enriched uranium.
SILEX is only one of a number of new approaches that have been investigated for uranium enrichment. The SILEX process [PDF] was developed by Dr. Michael Goldsworthy and Dr. Horst Struve.
As part of licensing the technology to the United States Enrichment Corporation, details of the SILEX are classified under the provisions of the US Atomic Energy Act. So while we don't have the whole story on the details of the process, it's reasonable to assume that only about three stages of enrichment are needed to produce five percent enriched uranium from ore, and only about seven stages to produce fully weapons-grade enriched uranium. Estimates suggest that a laser-based uranium enrichment plant would have an initial cost, size, and power requirement about one-fifth that of an equivalent centrifuge-based enrichment plant. The operating cost would also be expected to be far smaller.
Simpler, smaller, and less costly are characteristics that give laser enrichment of isotopes major potential to reduce the cost of nuclear power. However, these same characteristics also make such processes pose a substantial danger for widespread proliferation. Time will tell which potential future will win out, or if both hopes and concerns are valid.
Source: US Nuclear Regulatory Commission