Matter's mysterious twin, antimatter, has become slightly less mysterious, thanks to new research at the CERN particle physics lab in Switzerland. Scientists working on the ALPHA experiment, which makes, captures and studies antimatter atoms, have zapped antihydrogen atoms with lasers to measure their optical spectrum for the first time. By comparing the results with the well-documented spectrum of regular hydrogen, the team can check if antimatter behaves as predicted by the Standard Model of particle physics – and so far, it seems to.
The latest achievement is a progression of the ALPHA project's past successes. In 2010, the team was able to create, trap and study antimatter atoms for the very first time. The following year, they managed to keep those atoms stable for up to 16 minutes – a huge improvement over the previous average of less than a second. Given that extra study time, the team has now succeeded in mapping the spectrum of antimatter atoms.
Every element has its own spectrum, determined by which wavelengths of light they absorb and emit. Through the field of spectroscopy, scientists can then use that information for a wide range of applications, such as detecting the presence of certain metals in water or identifying the internal composition of distant stars. In this case, the team wanted to measure the spectrum of antihydrogen for the first time, and compare it to that of hydrogen, one of the most simple and best understood elements. According to the Standard Model, the two spectra should be identical, so any difference would have serious implications for our understanding of the universe.
"Using a laser to observe a transition in antihydrogen and comparing it to hydrogen to see if they obey the same laws of physics has always been a key goal of antimatter research," says Jeffrey Hangst, spokesperson for the ALPHA project.
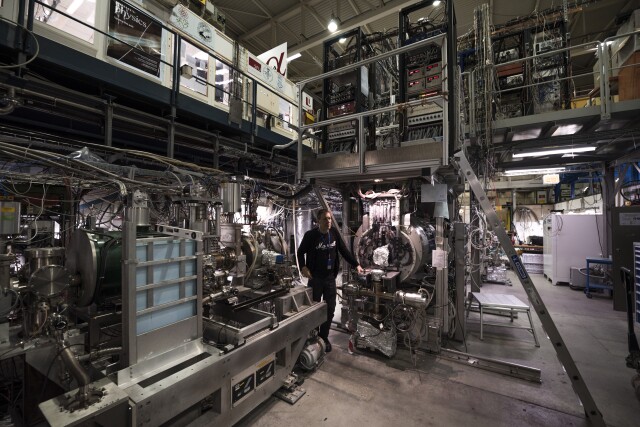
But antimatter is a tricky thing, and it raises a couple of other challenges that need to be overcome before its spectrum can be studied. The first hurdle is just getting it. Antimatter is incredibly rare, although nobody is quite sure why: according to the Big Bang theory, equal amounts of matter and antimatter should have been produced at the same time. Why our universe is now primarily made up of matter, and what happened to all the antimatter, are just some of the questions the researchers ultimately hope to answer.
Since it's so hard to find, the scientists have to make their own antimatter. This is done by mixing positrons with the plasmas from about 90,000 antiprotons, to create antihydrogen atoms. As difficult as this is to do, trapping the resulting antihydrogen poses the second major challenge. As antimatter atoms contain an opposite charge to their normal matter counterparts, if they ever come into contact with each other, both are immediately annihilated.
"Moving and trapping antiprotons or positrons is easy because they are charged particles," says Hangst. "But when you combine the two you get neutral antihydrogen, which is far more difficult to trap, so we have designed a very special magnetic trap that relies on the fact that antihydrogen is a little bit magnetic."
Only a tiny fraction of the antihydrogen that's created can be trapped, and only if those particles are moving slowly enough when they're created. The team produces around 25,000 antihydrogen atoms each time, and then stacks the atoms from two separate creation cycles together, which results in an average of just 14 anti-atoms trapped per trial.
Then, the scientists can zap those few remaining antimatter atoms with a laser beam tuned to a very specific frequency to study the spectrum of antihydrogen. By observing the so-called 1S-2S transition, the researchers found that, consistent with the Standard Model of particle physics, there was no difference between the spectrum of hydrogen and antihydrogen.
At least, that's the result that the current level of technology will allow. The ALPHA team plans to measure the spectrum more precisely in future, to put the Standard Model under further scrutiny and see if it continues to hold up.
The research was published in the journal Nature.
Jeffrey Hangst discusses the latest results from the ALPHA project in the video below:
Video credit: Jacques Herve Fichet/CERN
Source: CERN