Four tiny 3D organs connected themselves in a lab dish, forming a replica of the human pain pathway, in a new study. The discovery allows scientists to better understand chronic pain and how pain perception is affected by conditions like autism and offers an animal-free method of testing pain treatments.
Information about bodily sensations like pain, touch, itch, and movement gets conveyed from the periphery to the brain via the ascending sensory pathway. Disruptions to this pathway, caused by genetics or environmental factors, can lead to chronic pain or the sensory hypersensitivity that often accompanies autism spectrum disorder (ASD).
Researchers from Stanford Medicine have replicated this pain-sensing nerve pathway in 3D in a lab dish. This discovery improves our understanding of how we process pain signals and opens the door to the development of more effective pain treatments.
“We can now model this pathway non-invasively,” said Dr Sergiu Pașca, professor of psychiatry and behavioral sciences at Stanford University and the study’s corresponding author. “That will, we hope, help us learn how to better treat pain disorders.”
There is, of course, an added bonus to using these organoids, or miniaturized lab-made organs: scientists don’t need to experiment on sentient animals that feel pain.
“Their pain pathways are in some respects different from ours,” Pașca said. “Yet these animals experience pain. Our dish-based construct doesn’t. They transmit nervous signals that need to be further processed by other centers in our brains for us to experience the unpleasant, aversive feeling of pain.”
Pain signals originating in the body’s periphery are conveyed to the brain along a relay of neurons in four different parts of the ascending sensory pathway: the dorsal root ganglion, dorsal spinal cord, thalamus, and somatosensory cortex. The researchers used chemical signals to guide the growth of induced human pluripotent stem cells (hPSCs) into an organoid of each of the four distinct regions. The organoids contained nearly four million cells but were slightly less than one-tenth of an inch or 2.54 mm in diameter. The four organoids were placed side by side, and after about 100 days, the neurons in each had stitched themselves together to form what Pașca has termed an “assembloid.”
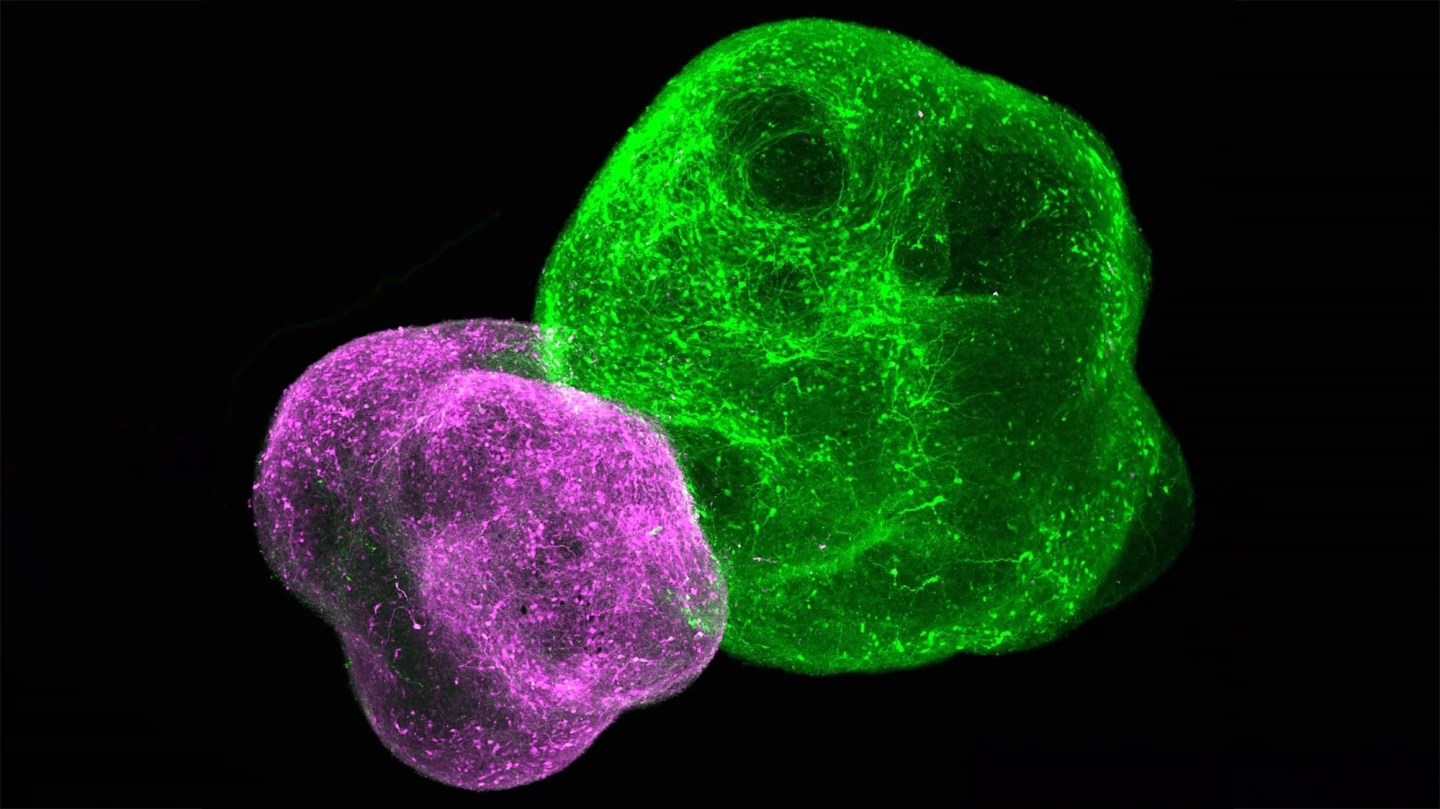
“We’ve been finding that we don’t need to know the details of assembly of these circuits as long as we make the parts and put them together correctly,” he said. “Once you put the organoids together, the cells find each other and connect in a meaningful way, giving rise to new features.”
The researchers saw that nerve activity spread along the length of the assembloid, mimicking what would happen in the human body: from the sensory organoid to the spinal organoid to the thalamic organoid and, finally, the cortical organoid. When chemicals like capsaicin – which gives chili peppers their heat – were used to induce pain, the wavelike activity increased.
“You’d never have been able to see this wavelike synchrony if you couldn’t watch all four organoids, connected, simultaneously,” said Pașca. “The brain is more than the sum of its parts.”
It’s already known that sodium channels play a critical role in the generation and conduction of nerve impulses that carry pain signals along a neuron’s membrane. Genetic mutations that affect Nav1.7, a particular type of sodium channel found predominantly on peripheral sensory neurons, can lead to either a debilitating hypersensitivity to pain or an inability to experience it. When the researchers created an assembloid with the mutated, pain-hypersensitivity Nav1.7, the mini-organ produced more frequent waves of spontaneous nerve transmissions from the sensory organoid through to the cortex organoid.
However, when they ‘switched off’ the Nav1.7 sodium channel in one organoid, they noticed that it still responded to the chemically generated pain stimulus by firing off signals, but the synchronicity between it and the other organoids was gone.
“The sensory neurons still fired,” Pașca said. “But they failed to engage the rest of the network in a coordinated manner.”
In addition to giving the researchers a greater understanding of how pain is transmitted, they say their assembloid could be used to study neurodevelopmental disorders like ASD, which can affect pain sensitivity and sensory stimulation in general. That’s because the assembloids, after only a few months of knitting themselves together, represent an early phase of fetal development. And, of course, the assembloids could be used to test pain medications.
“Nav1.7 appears to exist mostly on the surfaces of peripheral pain-sensing neurons,” said Pașca. “We think screening for drugs that tame sensory organoids’ ability to trigger excessive or inappropriate waves of neuronal transmission through our assembloid, without affecting the brain’s reward circuity as opioid drugs do – which is why they’re addictive – could lead to better-targeted therapies for pain.”
The study was published in the journal Nature.
Source: Stanford Medicine