The recent confirmation of gravity waves observed by the LIGO project represents a huge breakthrough in physics, verifying Albert Einstein's predictions regarding the effect of mass on space and time and supporting his general theory of relativity published in 1916. But what of his other grand hypothesis? Einstein's unified field theory consumed the last 30 years of his life without resolution, but how much closer have we come to a theory that brings every known force in the universe together into a single, all-encompassing frame of reference?
Today, the unified field theory has been modified and expanded to include scientific research unknown to Einstein, with the potential to affect our fundamental understanding of the universe and what we now know of quantum physics. We have learned a lot, but are these advances sufficient to take what we know of the macroscopic realm of gravity and merge it with the strange microscopic kingdom of quantum subatomic particles to create a Grand Unified Theory of Everything?
Building blocks
Before we tackle these questions, let's first take a quick look at the particles and forces at work in the natural world, how they interact, and why bringing them together in a theory that explains everything was (and still is) so daunting, and at the same time so alluring.
In the whole of the known universe, just two types of elementary particles are theorized to make up everything that exists – fermions and bosons. In simple terms, fermions are all the particles that make up matter (for example, electrons), and bosons are all the particles that carry force (for example, photons).
These elementary particles are then further defined by four fundamental forces that affect them: electromagnetic, strong nuclear, weak nuclear, and gravity. Each of these forces, or fields, exerts a particular influence on matter or energy.
The Standard Model of particle physics unites the first three of these forces in a single framework. It describes the interactions of the electromagnetic, strong and weak nuclear forces and all their carrier particles, as well as detailing how these forces act on all matter particles (including the recent discovery of the Higgs boson and its associated Higgs Field). In essence, the Standard Model is the logical extension of another much earlier unifying theory by James Clerk Maxwell that brought the electric and magnetic field theories together.
With the exception of gravity, each of the fundamental forces has its own corresponding boson type in the Standard Model; the strong nuclear force is carried by the gluon, the electromagnetic force is carried by the photon, and the W and Z bosons are accountable for the weak nuclear force.
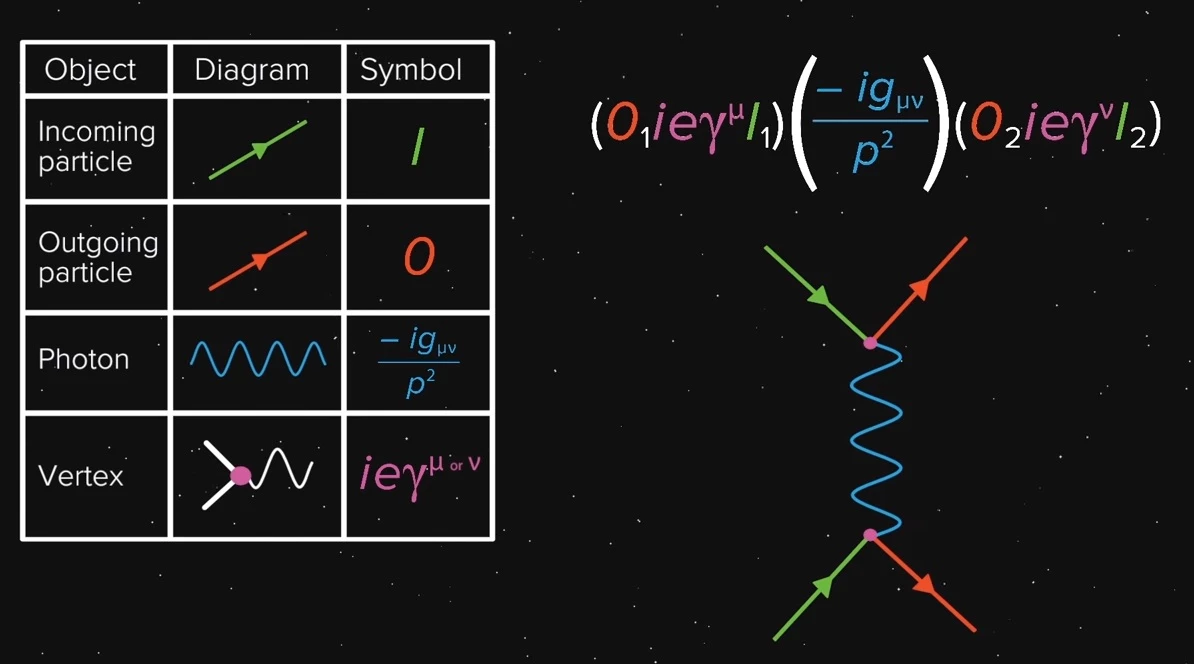
This means that the electromagnetic force influences particles with an electric charge, such as photons and electrons, the strong nuclear force provides the fundamental "glue" that holds atomic particles together – such as gluons and hadrons – and the weak nuclear force is defined by its ability to assist in the radioactive decay in elements – it is, for example, the force that initiates the nuclear fusion reaction that powers the Sun.
So, in essence, the Standard Model can be seen as the "Handbook to the Universe" for modern physics as it shows how the whole universe hangs together in every possible way.
Except, that is, for gravity.
On a grand scale – that is, at the macroscopic level – gravity remains the only one of the four "fields" yet to have clearly defined particles or adequately explained forces associated with it. And, though it is particularly strong around very massive bodies like planets, stars, and galaxies, gravity is the weakest of all the known forces at the microscopic level, where all the other forces exert their greatest influence.
So those in science that do believe all of the fields in the universe can be united in a Grand Unified Theory of Everything are looking for ways to expand the Standard Model to encompass gravity and show its interaction with other fields and particles to substantiate their theories. Some would say that the Standard Model is not so easily changed without substantial proof, and that proof has not been observed experimentally, so gravity cannot be included.
Alternatively, the Standard Model itself is may no longer considered a definitive version of all the fields in the universe because it is, they say, incompatible with the most successful theory of gravity produced to date; that of general relativity.
Regardless of which side of the theoretical divide physicists may sit, the idea that there could be a universal, Grand Unified Theory of Everything remains a captivating prospect, with the result that many proposals have been expounded over the years, and many researchers have spent a great deal of their time and energy pursuing this seductive scientific siren. Even the great Albert Einstein was not immune to its beckoning call.
Einstein's Theory
When Einstein first started his work in the 1930s on a unified field theory, he was ostensibly trying to reconcile his own general theory of relativity (which is, in effect, a field theory on gravitation) with that of Maxwell's unified field theory of electromagnetism. At the time, gravity and electromagnetism were believed to be the only two universal forces known to exist and Einstein's hope was to bring them together as different aspects of the same fundamental field.
At first glance, such a theory was a logical progression of his work and would make basic, rational sense of the things that we perceive in the universe. Such a theory would encompass a verifiable set of principles, constants, and equations that would describe the field for all of space and time and how it would behave when its parameters were altered. In effect, it would show how the whole of reality behaves in a rational, predictable manner that could be measured, tested, and verified.
However, dig deeper into the events of the time, and the motivation for Einstein to produce such a hypothesis as a purely logical progression of known physics may not have been quite as rational as it seemed.
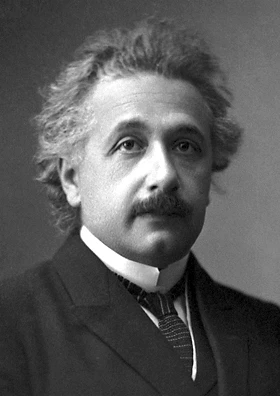
At a conference for the leading physicists of the day in Brussels in 1927, Einstein was involved in an infamous argument with the Danish physicist Niels Bohr about the newly-mooted field of quantum physics. Einstein himself had inadvertently set the wheels in motion for this new area of research when he published his 1905 paper (which won him the Nobel prize in 1921) describing the law of the photoelectric effect, where light was first described as a stream of discrete particles. However, he had not – and would not – leap to the conclusions Bohr and others drew from it.
Indeed, Einstein saw all the strange properties associated with quantum theory as proof of its flaws and dismissed it out of hand. Einstein refused to acknowledge a theory of the universe where Heisenberg's uncertainty principle put strict limits on how accurately one could measure the position, velocity, and energy of a particle. For Einstein, God did not "play dice with the universe" – he was a strict believer in the deterministic, reliable, repeatable nature of things in his scientifically-stable world, and random effects played no part in it for him.
To this end, Einstein sought to unify the forces at play in the universe from his perspective. And, indeed, from a macroscopic perspective, the forces of electromagnetism and gravity are alike in many ways and could be seen to exhibit properties that suggest they may have the same underlying forces at work. The measurable influence of both, for example, is inversely proportional to the square of the distance between two bodies, and both have a seemingly infinite distance over which that influence is capable of being seen or felt.
Einstein felt that he was right in this; he was sure that the universe operated in a rational, persistent, measurable way and he saw it as a way to prove once and for all that he was correct about the elemental deterministic nature of reality, free from quantum spooky actions at a distance. During a particularly rancorous period in 1935, he and a couple of other deterministic physicists published a paper purporting to finally prove the non-existence of quantum theory that was soundly rounded upon and howled down by many in the scientific community. Despite this, Einstein focused on little else for the rest of his life.
Einstein's opposition to the weirder aspects of quantum physics was probably part of the motivation for his quest and, ultimately, the reason that his theory never came to fruition as it stuck to macroscopic ways of looking at the universe. Indeed, trying then rejecting one mathematical model after another, Einstein continued to believe that everything – including quantum physics – could be explained when the two forces of electromagnetism and gravity were proven to be two products of the same fundamental force.
Einstein died on April 18, 1955. Next to his hospital bed were a dozen pages of paper covered in scores of notes and equations that had been crossed out, corrected, and re-written. To the very end, Einstein had continued to develop and explore the unification of these stubbornly disparate forces, never giving up his belief that his quest may be just one more line of mathematical code away.
Unifying disparate fields
Despite the fact that Einstein's unifying theory has never been supported by observations, let alone definitive mathematical proof, Einstein's work did ultimately lead many scientists to re-examine the universe in relation to a holistic theory of everything, including an amalgam of his gravitational theories and quantum gravity hypotheses.
Much work leading on from his theories provided tantalizing glimpses at possible gravitational interactions, including the behavior of the smallest of all fermions yet discovered – leptons and quarks.
This research led directly to the discovery of a gauge-invariant quantum field theory of the weak force, which included an electromagnetic interaction (and produced the "electroweak" concept that now shows correlation between electromagnetic and weak nuclear fields), which was, in itself, a great breakthrough in particle physics research. Unfortunately, however, it did not progress to include an observable gravitational component.
Nevertheless, buoyed by such revelations, theoretical physicists sought out a similar quantum field theory for the strong nuclear force, and eventually found one, dubbing it quantum chromodynamics. In this case, quarks are shown to interact through the exchange of gluons. This research has led to further postulations that the electroweak and strong nuclear forces could be united in a grand unified theory, which would then incorporate three of the four known forces in the universe. Again, however, an inclusion of the influence of gravity failed to be reconciled.
Of loops, strings and gravitons
So despite the successful conflation of the fields discussed above, physicists have been unable to formulate a complete particle-driven unified field theory for gravity since it seems to lack a force-carrier particle of its own.
There is, however, one contender: A contentious theoretical particle known as a "graviton".
The graviton moniker was apparently coined by the Russian physicists Dmitrii Blokhintsev and F. M. Gal'perin sometime in the mid 1930s (interestingly, around the time of the Einstein-Bohr stoush), in relation to the notion that if Einstein's predicted gravity waves existed, then they must also possess a quanta of energy, as does electromagnetic energy. That is, the electromagnetic and strong and weak nuclear forces all act through a "force carrier", which is exchanged between the interacting particles. These exchange carriers are also known as field particles, or gauge bosons.
The graviton, if it exists, doesn't seem to act like any of the other particles in the Standard Model, as it does not exhibit these force carrier behaviors. Put simply, unlike the other forces, gravity can not be absorbed, transformed, or shielded against, and it only attracts and never repels. In effect, this theoretical particle appears to possess no discernible way to interact with any other particle. This fact by itself would prohibit its inclusion in the Standard Model, partly because no instrument of sufficient size or efficiency could possibly be built to detect the supposedly tiny energies associated with it, but mostly because the entire concept runs into enormous theoretical difficulties at energies close to the Planck scale, which are the smallest sizes and energies able to be probed with particle accelerators.
Despite this, quantum gravity and other yet-to-be-proven quantum mechanical models such as string theory are often associated with gravitons, both of which rely on its existence. And though much hope is pinned on one of these theories eventually providing a unified description of gravity and particle physics, quantum gravity may prove the best contender. This is because string theory alone is not a physical descriptor of reality, but instead a self-contained mathematical model that describes all of the fundamental forces and the various forms of matter as models, not observed phenomena.
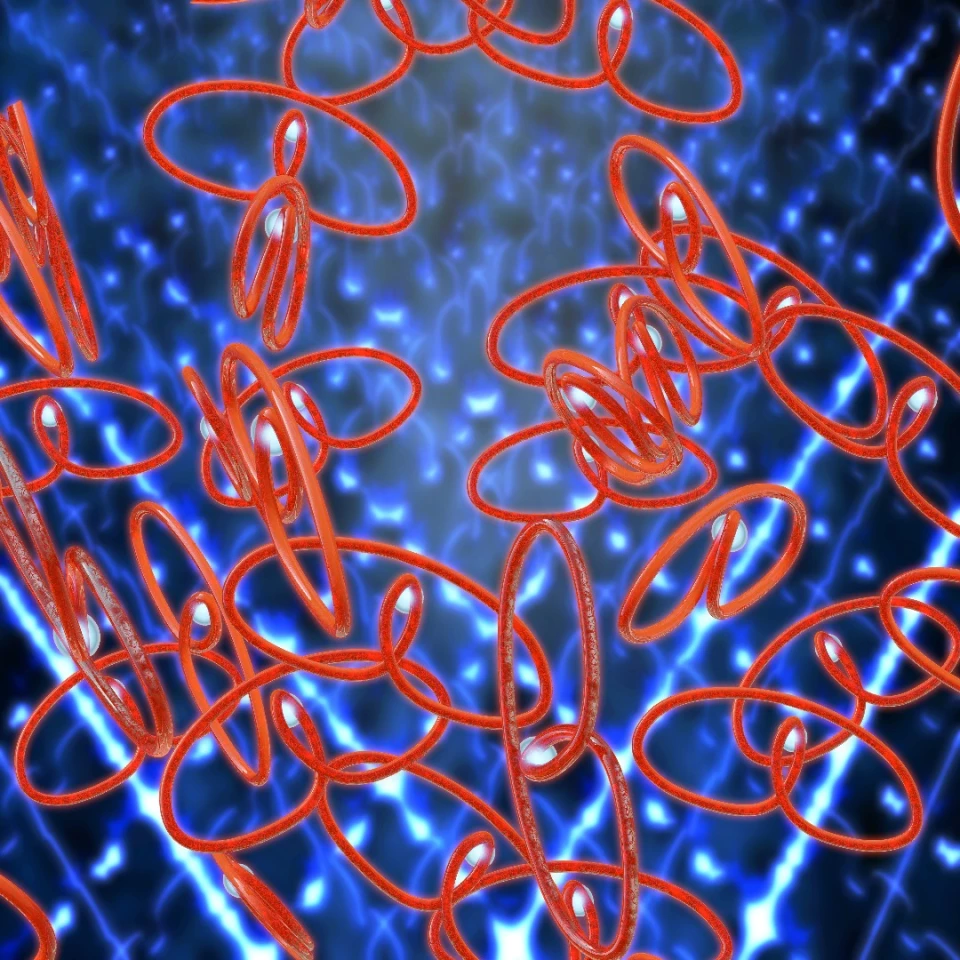
In string theory, the basic elements of the physical world are not particles, but infinitesimally-small, one-dimensional strings that vibrate at different frequencies, which supposedly give rise to all of the various particles and forces in the universe. The vibrating strings in this hypothesis need anywhere between 10 and 26 dimensions to perform their roles, depending upon the particular branch of the discipline applied.
Of all the theories posited to incorporate a fundamental explanation for all the forces so far found in the universe, loop quantum gravity is currently one of the more popular research areas. On the surface, it appears to be little more than a way to find a quantum theory of gravity based directly on Einstein's geometrical formulation, but its proponents claim it to be less unwieldy than string theory.
Essentially, loop quantum gravity hypothesizes that space-time actually consists of an infinite amount of small chunks. At a macroscopic level, these pieces appear to be one smooth sheet of time and space, but when you zoom in to the sub-atomic level, it actually consists of groups of dots connected by lines or loops. As such, space can be viewed as an extremely fine fabric or network woven of finite loops that provide an explanation for gravity, in that it predicts space, like matter, has an atomic structure.
The lack of observational evidence to these theories, though, does not preclude the notion that quantum gravitational effects may be somewhat more noticeable near black holes and, if this is so, then quantum gravitational fluctuations could theoretically leave their mark on the emission spectrum analyzed by LIGO and other gravitational wave experiments.
Given that the electromagnetic force between an electron and a proton in a hydrogen atom is 1039 times greater than the gravitational force between the same two particles, however, it will take one heck of a sensitive detector to go anywhere near differentiating such a tiny field from the background noise.
Barking up the wrong apple tree?
In all of these theories the inclusion of gravity in interactions with the forces and particles detailed in the the Standard Model, or some yet-to-be-proven quantum mechanical model, requires gravity to have some sort of particle (or equivalent force-carrier mechanism) of its own for any of these hypotheses to work at all.
And, despite Einstein trying to reconcile a Newtonian-style universe with that of his own General Theory of Relativity the central tenet of his hypothesis remains the idea that gravity is an effect of mass impinging on space-time, and no particle is identified or even required to make it work.
So, what if there is no particle. What is producing this "force"?
To help answer this, we have to look a little deeper into the mechanisms of mass creating curves in the fabric of space time around it. To make this a little more accessible, however, we will not use any of the mathematical formula behind it. Instead, we will use some (hopefully not too tortured) analogies to attempt to explain what is going on.
Imagine, if you will, that space is an enormous bowl of colored jello ("jelly" to those of you outside the US) and that each massive object in the universe is like a marble dropped into that gelatinous goo. Being solid, the marble takes up space in the jello and the jello itself is distorted around the marble. In very simple terms, this is a simple 3-dimensional interpretation of what space would look like if we could see its distortions; a gelatinous, amorphous substance that contains lumps of matter that it molds itself around, but which distort it at the same time.
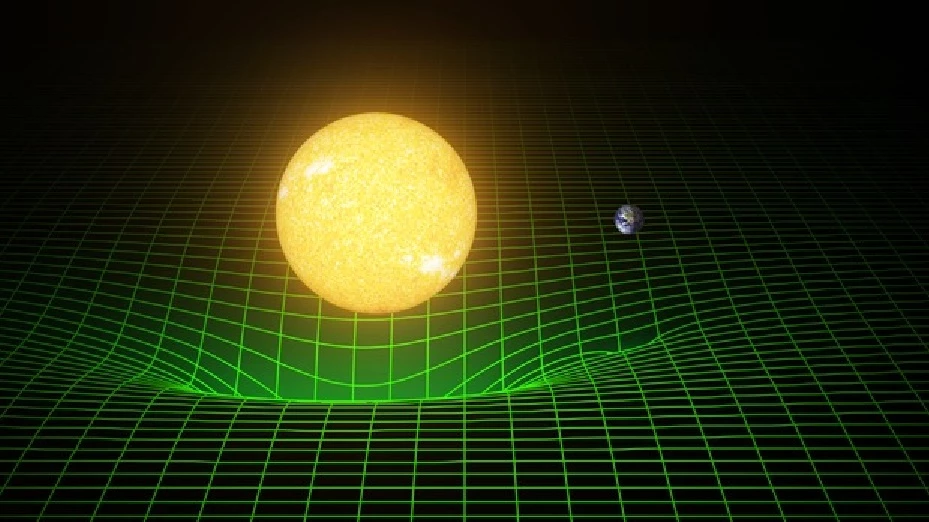
Now take this analogy a little further, and we see that small particles do not distort the jello of space very much, but the more of these particles you bring together into larger masses, the greater the effect they have in producing distortions. Kick this analogy up a notch, and picture this collection of marbles being able to spin themselves and move forward through this gelatinous substance. All around the marbles, the glutinous space-time material would be contorted, with slow-moving vortices creating voids of jello in their wake as they pushed through it. These distortions and voids are the (very simplified) equivalent to mass creating curvatures in space.
In the version of space imagined by Isaac Newton, with no forces acting between objects they tend to move along the straightest possible line at a constant velocity. In this very basic concept, Einstein's special relativity and Newton's classic mechanics agree. If these objects deviate from their path and move towards each other, Newtonian theory would have it that some force has acted one or both of these objects. And this is exactly what Newton's theory of gravity dictates; that a force, such as a nearby massive object, has caused the objects in question to move towards it. That is, Newton thought that a mass exerts force directly onto nearby objects.
But Einstein realized that gravity is actually a product of the curvature of space where any objects close to a large mass have their ordinary straight line motion curved, giving the appearance of being attracted to the body in question. That is, just as a straight line following the surface of a sphere isn't really straight, then so too perceived straight line motion of an object near a mass is actually curved by the distortion that mass impinges on space-time. As a result, small masses near to a massive sphere fall toward the distortion in space that the mass has created (just as in our jello analogy). But this space-time distortion effect we call "gravity" doesn't move these masses from their straight lines, it simply redefines in relative terms what moving in the straightest possible way near a mass means.
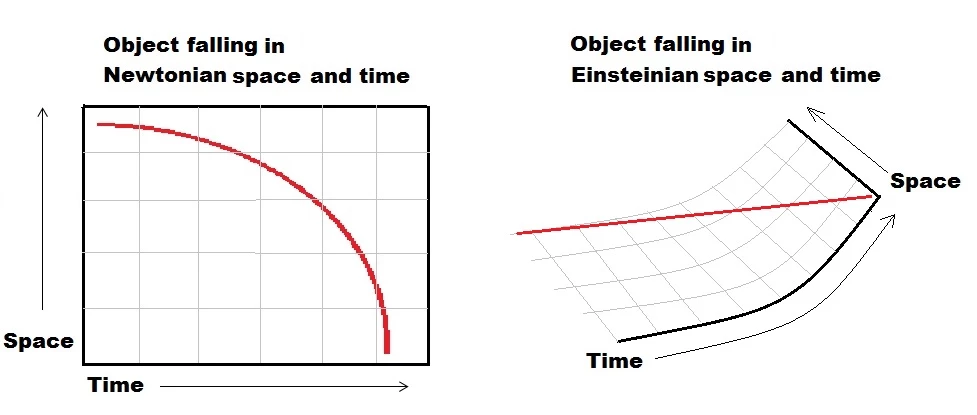
Using yet another analogy, imagine that you are travelling in a train on a straight track on a flat surface at night. Riding in the carriage, everything feels in equilibrium; you are moving forward horizontally, in a straight line, and with very little apparent motion. Now imagine that your train reaches a point on the line where it appears to dip into a steep, downward incline.
Your train continues on its straight-line journey on its tracks, but that motion now curves downwards into the valley below. You can neither see the curve nor (if the driver is not so reckless as to speed into the dip) can you feel the change. But change you have. Your train is now traveling in a downward curve that appears to be in all respects to your senses a straight line, but is in fact distinctly bent.
Now imagine that the train is a small mass, the train track is the apparent straight-line motion of objects through space, and the steep incline is the curvature in space-time created by a large mass. In this way, any object that appears to be traveling forward in time and space in a linear, straight-line motion as observed from your own point of view, is in fact, following the curvature of space-time created by a massive object and giving the illusion that the driver of this motion is the force of gravity.
As such, gravity is not actually exerting a force, because it is not a force itself – it is merely an effect of the distortion of space-time being observed as something that has the apparent behavior of a force, but where objects are merely following the curve created by a mass.
And this is all based on the fact that – and I am repeating this because it is worth doing so – gravity cannot be absorbed, transformed, or shielded against, and it only attracts and never repels, and so it displays no aspects at all comparable to other forces in the Standard Model.
Is it all too hard?
So, if gravity isn't a force as such, then what is the thing that mass does to space-time to make the effect of gravity appear so real? And will this gravitational effect then be able to be tied into a Grand Unified Theory of Everything, if we can work out what makes mass and gravity so intertwined?
One part of the answer to all this may come with the recent discovery of the Higgs boson using the Large Hadron Collider at CERN.
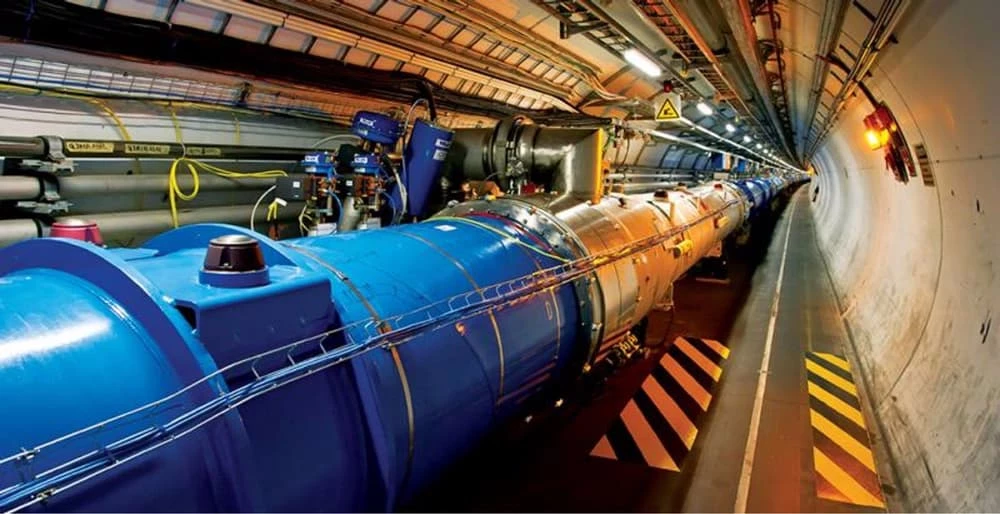
Now included as one of the particles in the Standard Model, the Higgs Boson acts like other bosons, in that it interacts with particles to transfer force. This process starts with the energy of a gauge boson interacting with the Higgs field in the form of kinetic energy as it moves through the field.
Interacting with the Higgs field slows down the gauge boson, thereby reducing the amount of kinetic energy which is then converted into mass-energy, and this produces a Higgs boson. The Higgs boson, then, is the force-carrier that imparts mass to particles that travel through its energy field.
So, now that the connection between bosons and mass has been made, then there should, ipso facto, be a relationship between that mass/force co-existence and the amount of influence exerted on space-time and the resultant gravitational effect. In other words, now that we know the mechanism behind the creation of mass, we should also be on the road to understanding how an increase in mass affects a proportional increase in gravity.
However – and this is the rub – if gravity is a side-effect, and not a force, then it cannot possibly possess an equivalent force-carrier particle and, therefore, is irreconcilable with the other particles and fields in the Standard Model.
But more than this, the real problem in postulating a theory of everything may simply be that it is so exceedingly difficult to identify the ultimate, fundamental, mechanisms at work in the universe because their interactions are at such infinitesimally tiny levels of energy and mind-bogglingly picayune scales that we are simply unable to detect anything other than their cumulative effects in the carrier medium of space-time. After all, it took the collision of two massive black holes to produce gravity waves of sufficient strength to produce a distortion in space-time that moved detecting laser beams on LIGO a fraction the size of a proton.
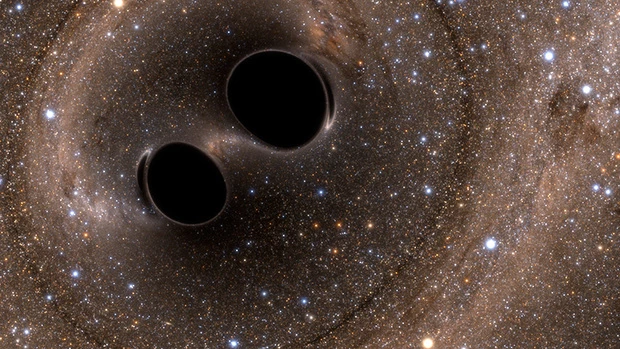
So, given all of these reasons for gravity to not be included in a unified theory of everything, is there any hope for a theory that at least comes close? Provided that the definition of "everything" is a field theory that encompasses the particles and forces that have been made a verified member of the Standard Model with provable physical attributes and corresponding measurable forces, then the answer would appear to be a tentative yes. But any attempt to include gravity without definitive proof of a force-carrier mechanism in such a theory may simply be to replicate a never-ending folly. It is, after all, a conundrum that even the great genius of Einstein was unable to solve.