Some fusion projects aim to create hundred-million degree working temperatures in magnetically confined plasma. The CEO of TAE Technologies tells us his team's aiming for 10 times that temperature, targeting cheaper, easier and safer boron fuel.
If you bang the nuclei of two atoms together hard enough, they can fuse together to create a different element. If you use the right elements, the resulting fused atom will weigh less than the two you've banged together to form it, and the difference in mass will be released as energy, as predicted by Einstein's famous E=MC2 equation. C2 – the square of the speed of light – is a rather large number, so a small mass of fuel can produce a large release of energy.
The problem is, atomic nuclei are extremely tiny – and positively charged, so they repel one another, making it extremely hard to bang them together in the first place. It happens all day long in the Sun, producing the energy that warms the solar system, but then the Sun's got colossal gravity as well as super-high temperatures on its side, respectively pulling atoms toward the middle of its core, and making them vibrate with such ferocious energy that they randomly strike one another and fuse together, releasing yet more heat. The conditions are perfect to create constant fusion reactions that will continue for billions of years.
People have been trying to replicate this process on Earth for many decades, drawn by a promise of abundant, clean energy that's even safer than nuclear fission (which itself, despite a few high-profile incidents, remains one of the safest forms of energy yet known to man). Nobody's got a fusion plant working yet, but progress in the field seems to be accelerating.
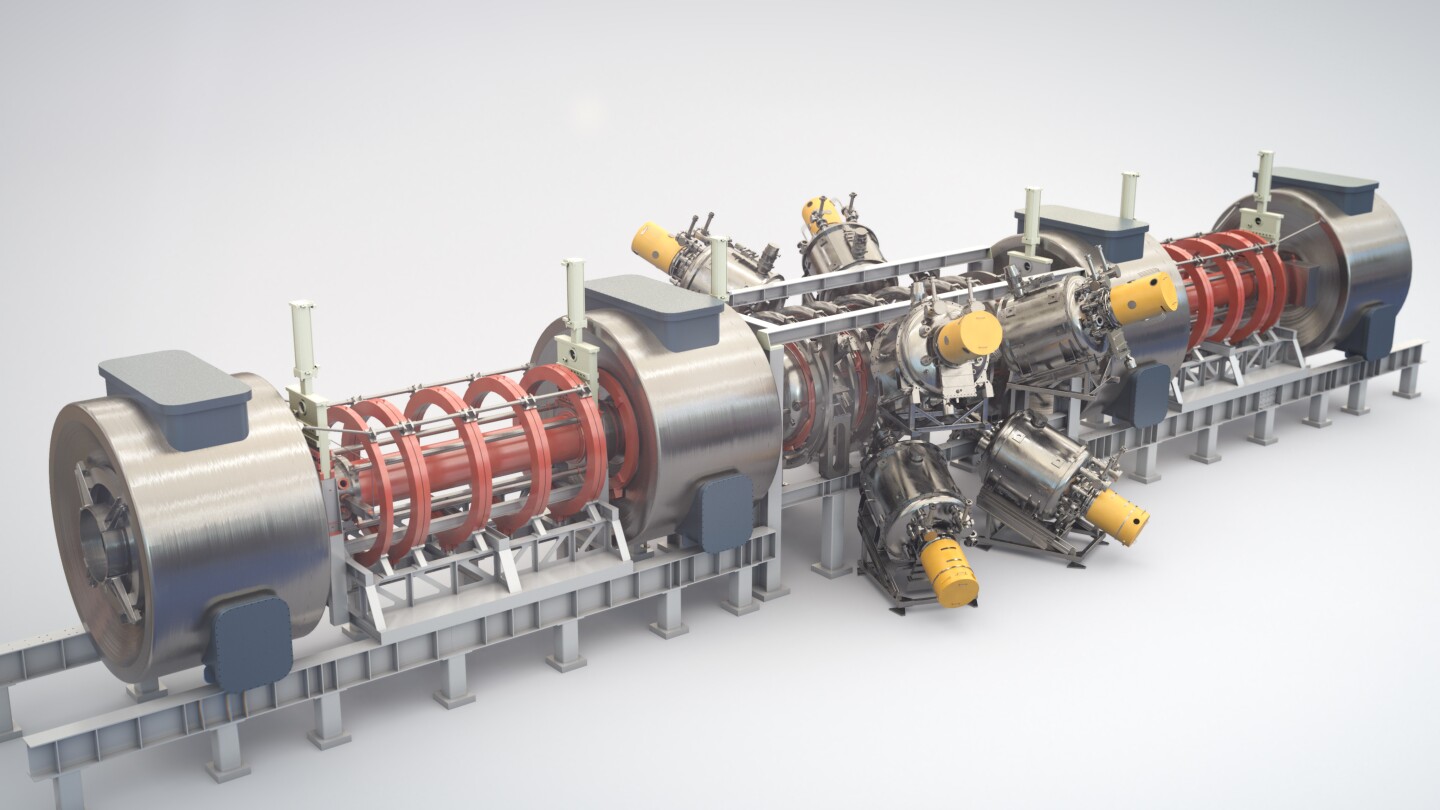
Without the benefit of the Sun's enormous mass and gravitational pull, many Earthbound fusion efforts rely on even higher temperatures. In magnetic confinement fusion projects, plasma is superheated to somewhere over 100 million degrees Celsius (180 million °F) – nearly four times hotter than it gets in the core of the Sun. Heat is movement at the atomic level, and this additional movement is expected to smash atoms in the plasma against each other hard enough to overcome nuclear repulsion and start fusion reactions.
This, mind you, is assuming you're using tritium and deuterium as fuel. That's what the massive, international ITER project is doing, for example. But tritium comes with its own issues, including the fact that it's radioactive, and thus will irradiate materials used in a reactor. It's also rare; there's only about 25 kilograms of it stockpiled around the world today, and by its own estimations, ITER reckons it's going to use almost all of that in its experiments. It's more complex than that, as explained in this article in Science, but its scarcity and radioactivity will make fusion power much more expensive.
"Practical constraints immediately become cost constraints," Michl Binderbauer told us over a video chat. Binderbauer is the CEO of TAE Technology, formerly known as Tri Alpha Energy. This California company, founded way back in 1998 as a spinout from UC Irvine, has rallied more than US$1.2 billion around its cause, from investors including Google, Chevron, Goldman Sachs, Paul Allen, the Rockefeller family, and others. And it's used it to build several prototypes, gradually increasing in size and scope.
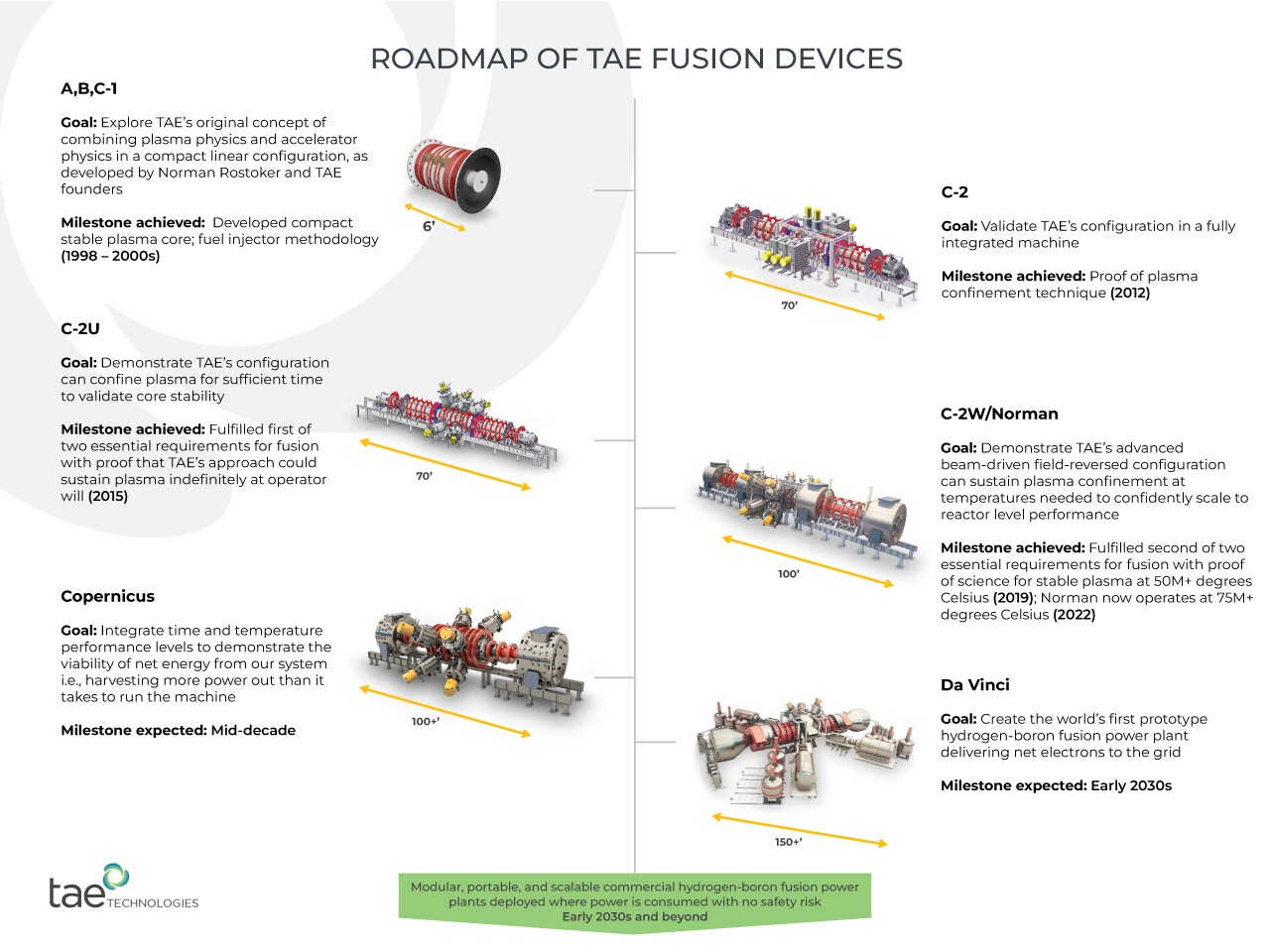
The fifth, named Norman, was completed in 2017, and designed to target plasma temperatures of 30 million °C (54 million °F). Norman has performed much better than promised; TAE has demonstrated it's capable of sustaining plasma at 75 million °C (135 million °F), placing the company ahead of schedule. But it's not targeting 100 million degrees. It's targeting a billion degrees.
What TAE really wants to achieve is hydrogen-boron fusion. "It has all the benefits that tritium lacks," said Binderbauer. "There's no radioactivity involved in the input or the output. The output is helium, chemically inert, about as benign as you can get. Boron's made by the metric ton today. It's used in detergents, it's a commodity product. So there's no scarcity, it's found everywhere; there's no sort of Saudi Arabia of boron."
The drawback is that boron is a larger atom than tritium, with more positive charges at its core, so in a magnetic confinement design you need much, much more energy to fuse it. A billion degrees' worth of energy. "People have shied away from boron historically because fusion is already darn hard," said Binderbauer. "But it turns out that if you design your fusion reactor around tritium, you get some serious defects, along with a big disconnect from the commercial world where cost is important."
In order to achieve temperatures 10 times higher than anything the tokamaks of the world are even aiming for, TAE had to design a very different reactor. So it did, inspired by particle accelerators at CERN. "That was [Founder] Norman [Rostoker]'s absolutely ingenious insight," said Binderbauer. "CERN can accelerate soups of particles to astronomical conditions. Their marker, self-reported, is five trillion degrees, or the equivalent. We only need a billion. Can we harvest some of the building blocks of those principles and translate those into fusion? He thought we could."
CERN can accelerate soups of particles to astronomical conditions. Their marker, self-reported, is five trillion degrees, or the equivalent. We only need a billion.
By the late 1990s, the team had done enough theory, modeling and computational simulation to get going on some physical prototypes. "That became a bit more difficult than I felt it would initially," said Binderbauer. "We've ended up on a journey that's now 20 years plus. And it's been a journey much less about scientific discovery than about technology, and engineering components that can do what we're asking of these machines."
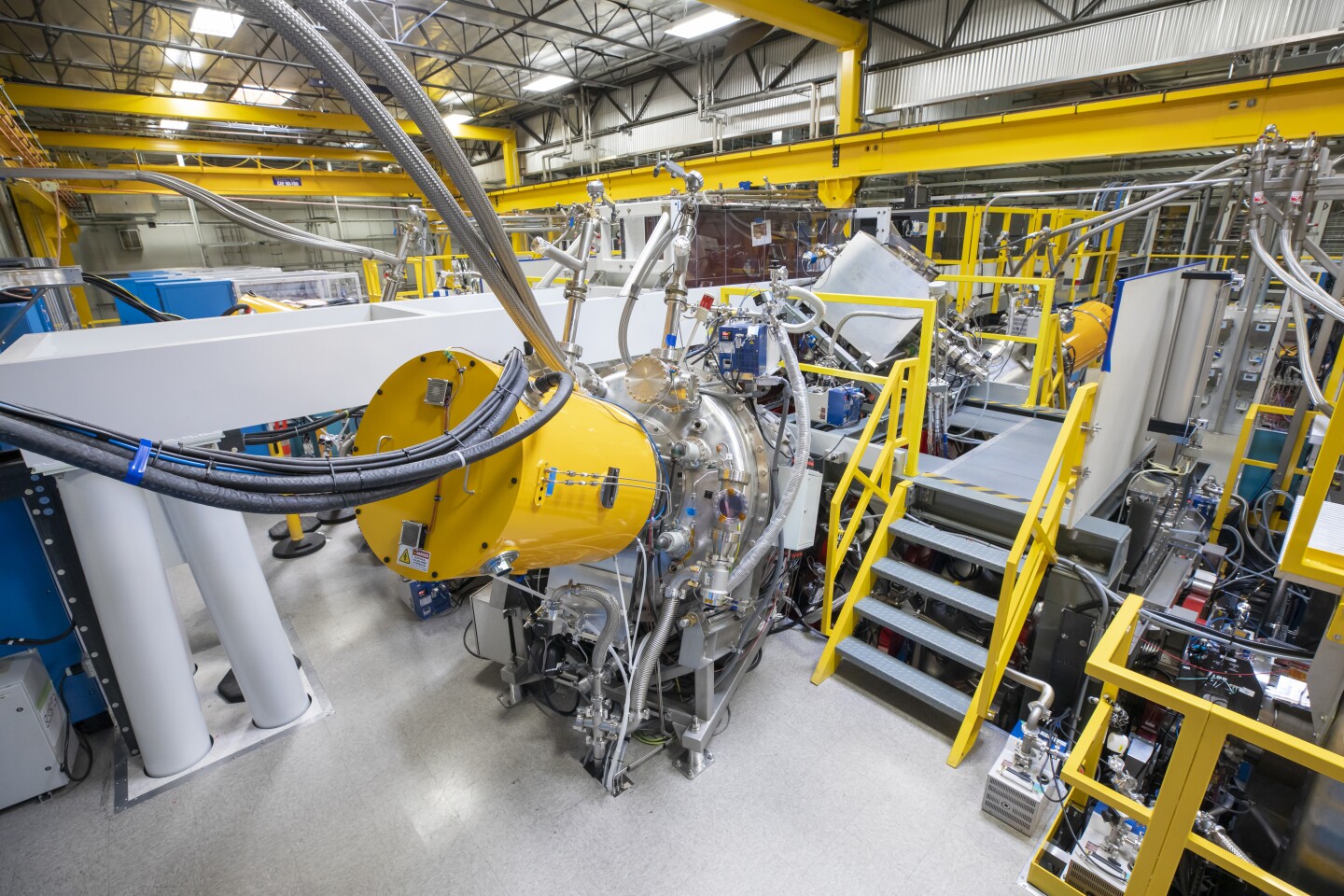
TAE had to develop its own particle accelerators, protruding like a matador's banderillas from the central, cylindrical tunnel of the company's unique reactor design. "You can think of these like fuel injectors on a car's engine," said Binderbauer. "They bring very high energy particles into the core of our system and create these very high energy elevated temperature conditions."
Where the Large Hadron Collider's particle accelerator is a 27-km (16.8 mile)-long ring, TAE's are remarkably compact. "Compared to CERN, ours are a joke," continued Binderbauer. "They can create trillions of degrees, we need a factor of 1,000 to 10,000 less. They're accelerating more or less single particles up to 99.999% of the speed of light, in a vacuum system. We need to push a lot more particles, at lower energy and speed. So ours are a lot more modest in terms of physical scale. But they do run at much higher current. Instead of milliamps and microamps, we're producing hundreds of amps.
Rather than running its plasma around in a donut shape, TAE keeps it spinning in place, confined by powerful magnetic rings. "They're simple geometry magnets," explained Chief Marketing Officer Jim McNiel. "There's nothing interlinked. We make plasmas that look like ellipsoids, rotating inside the cylinder along the axis of the cylinder. We can control the rotation, it's actually rather elegant and simple. You may remember playing with a spinning top as a kid – when it slows down, it becomes unstable, but if it rotates at the right rate, through gyroscopic stabilization it's very steady and predictable. We're doing the same thing here, we control the rate of rotation and it sits happily in place."
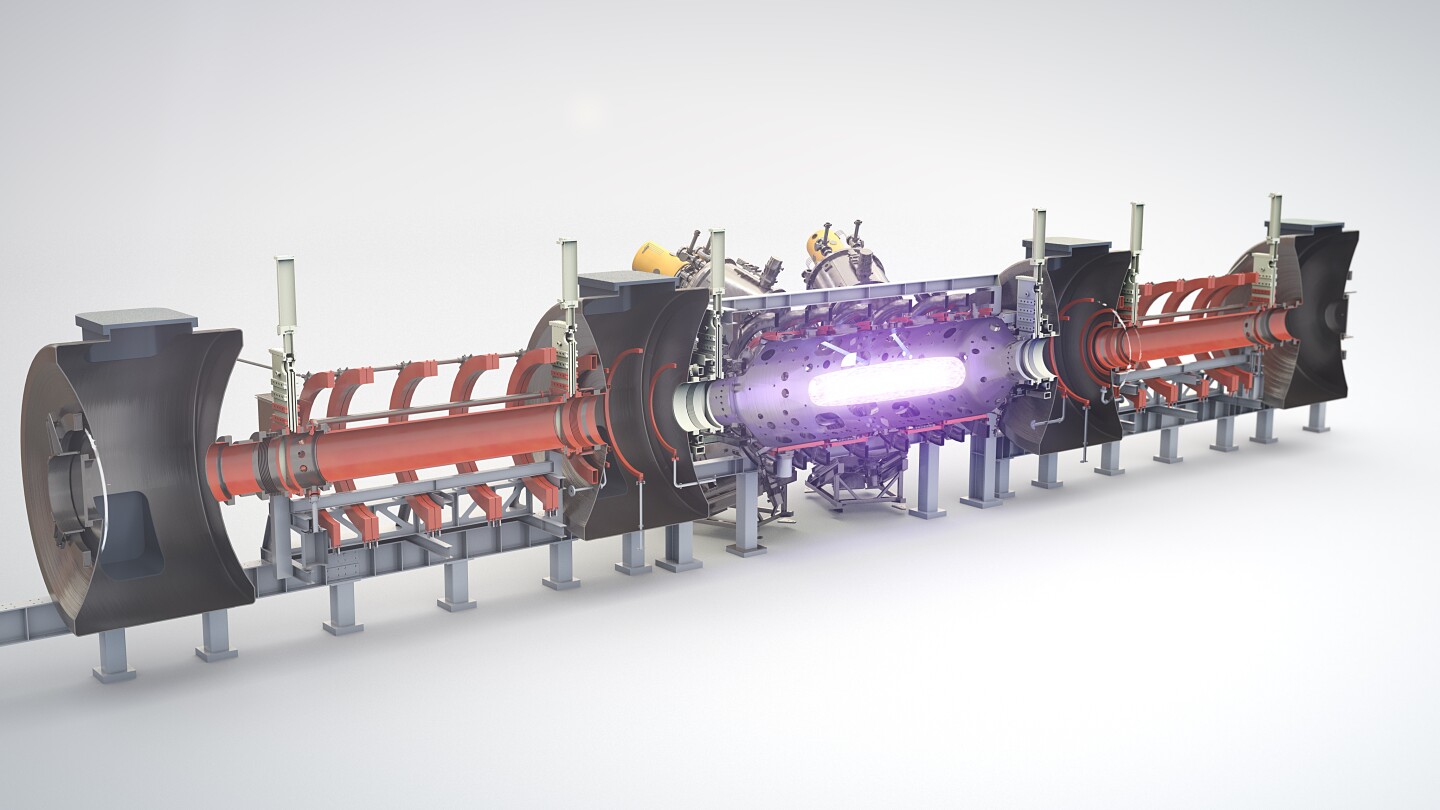
In that sense, the team says it's more or less like a straightened-out section of a tokamak donut, with the benefit that magnets can be easily removed or replaced without having to take the whole thing apart, and TAE can run huge field divertors at either end of the tube, acting like trash cans to absorb impurities and exhaust particles. "In a tokamak, these are huge engineering hurdles," said Binderbauer. "They have to live inside the magnetic cocoon. That's precious real estate. So you have to make them tiny, but they have to absorb the same amount of heat as we do, with a very small surface. Our current machine, Norman, runs divertors about three meters in diameter, made out of simple sheet metal. And you know, if we find ourselves having a heat transfer problem, we can just double the size."
"And in terms of magnetic efficiency, which is a basic number you can get from looking at the geometry and design of the reactor, that's about 10% in a tokamak, and about 90% in a design like this," he continued. "Magnets are a big, expensive part of these fusion systems, so if you can make efficient use of your magnets, you can generate lower-cost power. There's absolutely no question that it's lower cost."
With the fifth-generation machine over-performing to the tune of 250% of its design goals, TAE is beginning to find that the hotter its plasma gets, the better its particle accelerators and magnetic confinement systems perform, with lower rates of particle and heat leakage. "We did expect that, but it took us 10 years, and a lot of technology development to actually show that," said Binderbauer. "But over five generations of prototype we now have the data sets to show that the system behaves better as the temperature rises. So we have a high degree of confidence that we can do this, not only up to 150 million degrees for tritium conditions, but to a billion degrees and beyond for boron. That'll be late in the decade, or early 30s."
The next prototype, the sixth-gen Copernicus system, is designed to hit those 100-150 million degrees to prove it has the capability to create tritium-relevant conditions. "We have a pretty good team that's built these large machines on time and on budget for the last three generations," said Binderbauer. "But with COVID and supply chain issues, there are some additional challenges we haven't faced before. But being humble about it, I predict that somewhere around 2025 we'll have this machine huffing and puffing and producing the data we hoped for."
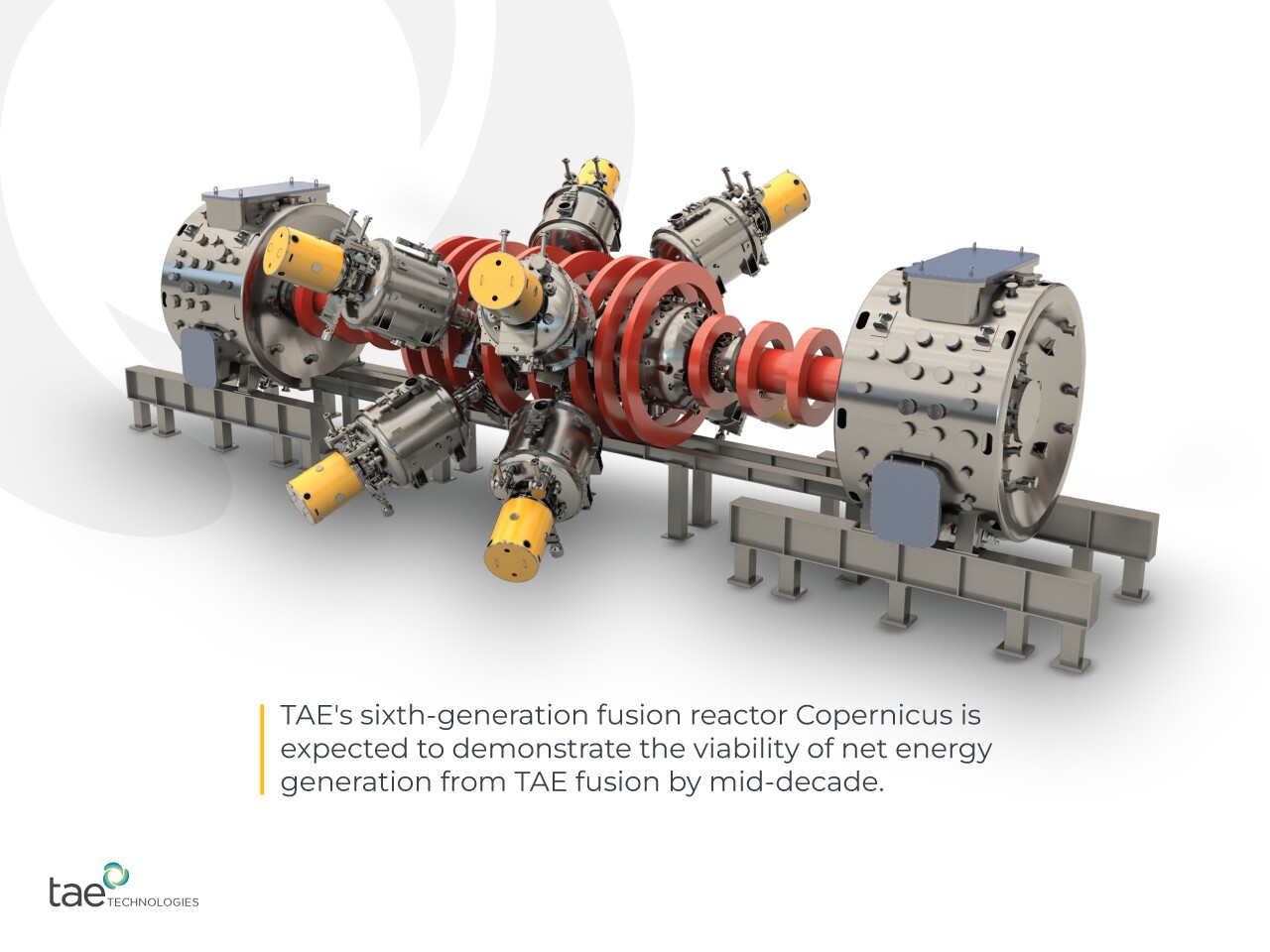
Copernicus is designed to demonstrate a positive energy balance – a Q factor greater than one, meaning that it generates more power than it uses. It'll be hooked up to sensors, though, rather than an electricity-generating back end. "If and when that succeeds, we'll move on to the final step, the Da Vinci machine, which will take this up to hydrogen-boron pB11 conditions," said Binderbauer. "We're doing a lot of modeling on this, and we're getting more and more confident we can actually do this. But it's another big step, to be clear!" The Da Vinci machine will be a prototype power plant, running a steam-turbine generator that connects to the grid.
The patience required of TAE's investors is enormous in today's world. These machines are cutting-edge, and expensive, and the commercial payoff is way over the horizon. The company's approach is to work on a money-by-milestone model, each stage targeted at reducing the biggest investment risk possible for the least money. "We have an independent science panel, composed of some of the brightest people in fusion physics," said Binderbauer. "They don't have stock holdings or any interest in the company. They come twice a year, they're paid for their time, and they critique our work, and give an independent validation of whether we've achieved a given milestone."
Relieving some of the pressure on investors, TAE has spun out commercial entities based on the technologies it's developed. The particle accelerators, for example, have been repurposed as targeted radiation therapy devices for cancer patients. The power staging and distribution systems that synchronize the operation of 80,000 electrified parts with high precision, is being used to develop better and more efficient EV drivetrains.
"So yes, the returns don't come overnight, but these technology spinouts create more of a hedged risk profile," said Binderbauer. "Early on, we relied on angel investors and philanthropic billionaires, but now we're bringing in people like Google, Sumitomo, Chevron, who see strategic opportunities for themselves in the evolution of the technology base as well."
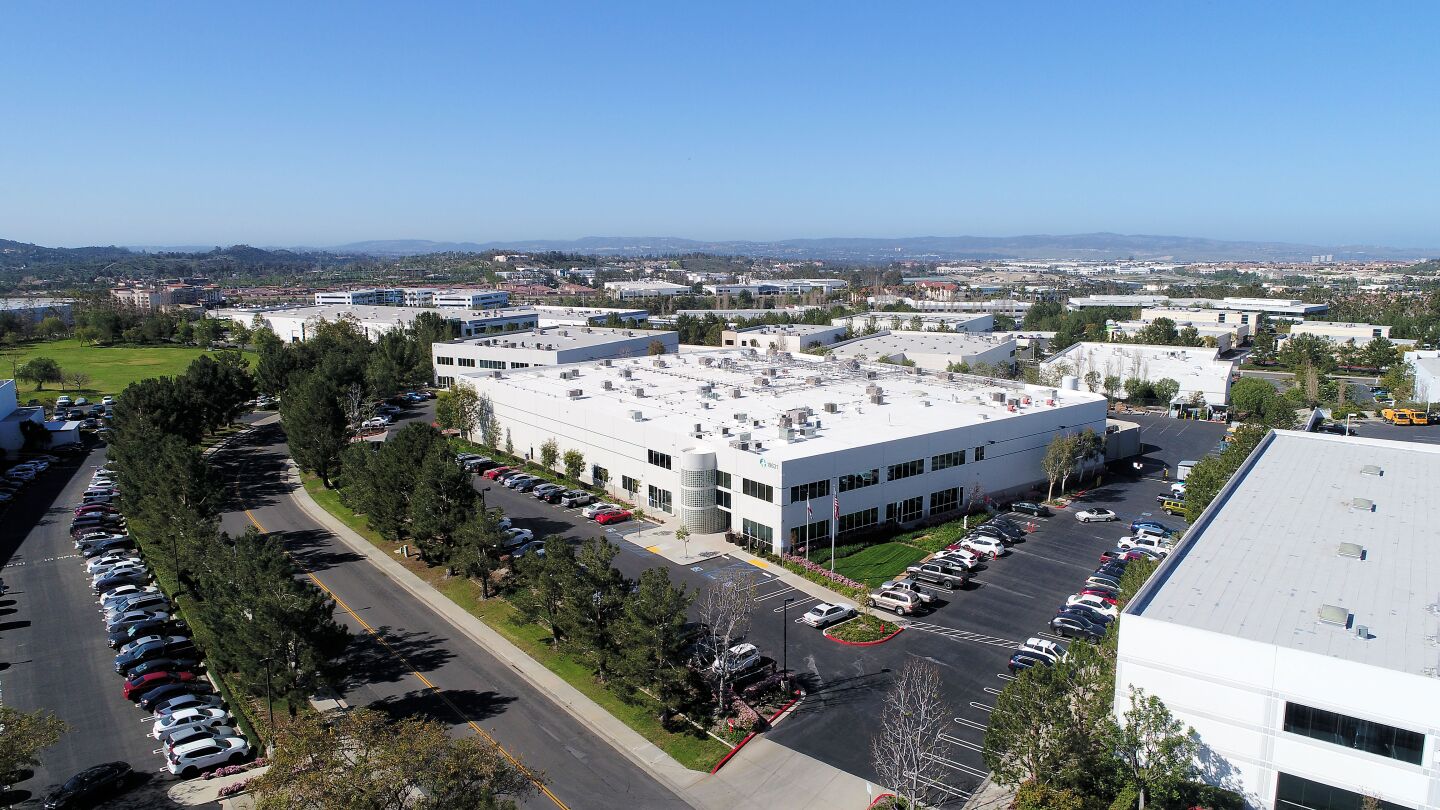
On the other side of the investment risk is the potential reward – ownership in a clean energy technology that uses abundant, simple, cheap fuels, and that can respond to demand surges by leaping from ambient temperatures to a billion degrees in a few thousandths of a second. So the power can spool up as soon as the water's hot enough to spin the turbines.
On that last point, the team says it's got a small number of staff working on a next-gen direct energy capture system that would take the big, inconvenient turbine out of the system altogether and replace it with a totally solid-state energy capture device loosely related to solar panels.
"That's another generation beyond, but what comes off the fusion reaction is basically intense light," said Binderbauer. "Soft X-rays to be specific, the same kind they use to do your teeth exams. So we're looking into a direct capture system that would work a little like solar cells on steroids. There are some very cool opportunities there, they can theoretically be much higher efficiency than solar cells. The X-rays will free electrons in an absorber material, and those will go straight to the grid as DC electricity. This is benchtop-level exploratory small-lab stuff for us, we've got to get the core working first, but it's a sincere effort, and it's one of a few different ideas we've got for beyond commercialization. It'd bring the efficiencies way up, and the costs way down."
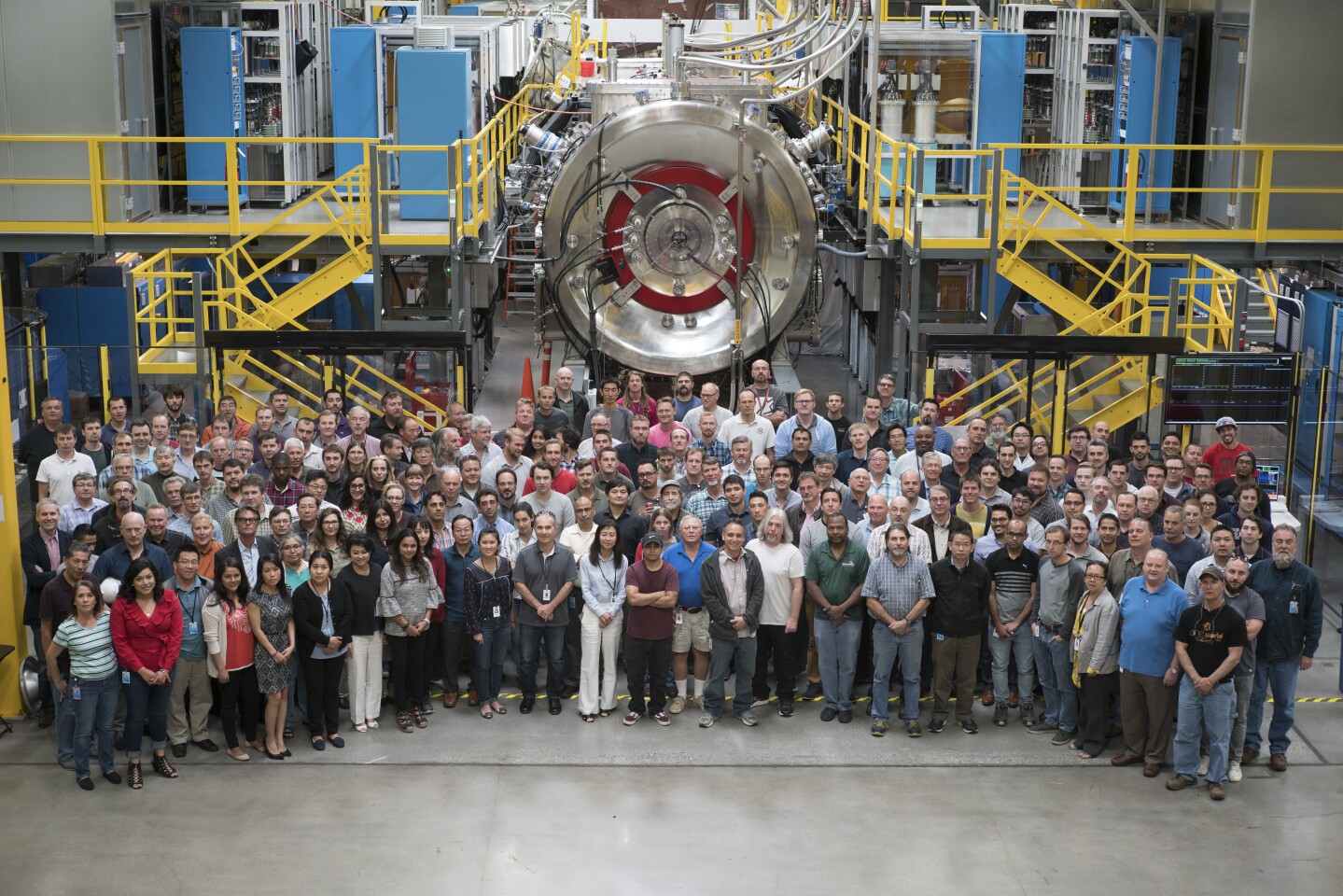
What indeed will the costs look like for an early commercial hydrogen-boron reactor? After all, if we assume everything goes according to plan, these things will enter the energy market in the early-to-mid 2030s, to prop up power grids based increasingly on cheap renewables, along with a range of short- and longer-term energy storage and release designs that kick in when renewables aren't meeting demand. Does TAE have an estimate on fusion's levelized cost of energy? Can it compete with big batteries?
"I'm willing enough to share that, because they're actually not so bad," said Binderbauer. "I think they'll start out somewhere in the midfield of today's generation's spread of costs. In the US, gas-fired electricity can be done in the one- to two-penny per kilowatt-hour range. Nuclear, particularly high-safety nuclear, is more expensive, maybe 10-15 cents. Our first-generation plant, we expect to come in around the 6-7 cents per kilowatt-hour region."
"Yes, there's still some guesswork involved," he continued. "But we've got a pretty good sense of what we need to build, and we've worked with sophisticated supply chain partners to estimate costs on these plants. I can tell you that number because we believe the cost isn't vastly off. That's without carbon credits or subsidies, and that's our first plant. From there, prices will come down. Maybe we'll never be the cheapest source, but we'll be practical, completely green, low resource intensity. We can run safely in high-density urban centers all over the world."
Safety is one of fusion's key advantages over nuclear fission, but the team says it's still a hurdle for them every time somebody hears their reactor's planning to run nearly 40 times hotter than the core of the Sun. "Public sentiment is not to be underestimated," said Binderbauer. "In fact, it may be one of the biggest unmet needs. Elected officials take their input from their constituents. And I get that question all the time: a billion degrees, isn't that going to melt the reactor and burn a hole in the world?"
I get that question all the time: a billion degrees, isn't that going to melt the reactor and burn a hole in the world?
In short, no it won't. Remember, CERN has already created temperatures over five trillion degrees without incident. "People need to understand just how small atoms are, and how quickly that heat diffuses," said McNiel. "There's eight and a half octillion atoms in a 150-pound human. So we've got a small, low-density cloud of them at very high energy, suspended and spinning in a magnetic cage. If they come into contact with anything that's material, they lose all their energy instantly."
"If you take our 75-million-degree plasma, and you put an ice cube out of your fridge in, guess what happens?" said Binderbauer. "The ice will turn to water, but it won't even heat up. It just phase-changes. But this is not clear to most people at all. All the investors ask these things, multiple times. Likewise, our particle accelerators, they're high-intensity beams. If you fired one at a steel plate, it'd burn a hole through it in a second. But there are ways to control these things, and they shut down."
"There's no thermal runaway. There's no core meltdown. With hydrogen-boron, there's no radioactivity," he continued. "The risk profile is safer than fission, there's just no question. But the entire industry is engaged today trying to figure out the right regulatory framework. What's fair, what can make the public feel safe. Last year, the UK enacted fusion regulations already – they decided it's not like fission and needs a different regulatory framework. But that's still in play in the USA. The Nuclear Regulatory Commission has been holding hearings for the last year-plus, and there's still a debate as to whether a fusion plant will have to go through the same rigorous process as a new fission plant design. We certainly hope they'll treat it differently, but there's no guarantee we'll get that."
If fusion is treated the same as fission, the lengthy, complex approval process could add 10 years' worth of red tape to the wait for commercial fusion energy, along with a commensurate increase in price. So the entire industry is hoping governments and regulators get their heads around this unique form of clean energy, fast, before the rubber starts hitting the road on commercial fusion – not in 30 years' time, but perhaps as soon as the early 2030s.
Hear Binderbauer explain more in the video below.
Source: TAE Technologies
Editor's note: this piece was updated August 13 in response to clarifications from the company.