Researchers have developed a 3D-printable electrode that looks like a single strand of human hair and measures brain activity more reliably than the current method used to diagnose things like epilepsy and sleep disorders.
When you imagine someone having an electroencephalogram (EEG) to diagnose a condition such as epilepsy, for example, you probably picture them with a head covered in electrodes. That’s because the standard EEG typically uses 21 of them, affixed to the scalp in strategic positions to capture activity from various brain regions.
However, a team of researchers from Pennsylvania State University (Penn State) has ushered in the future of EEG, developing a single electrode that looks just like a strand of hair and is more reliable than the standard, multi-electrode version.
“This electrode allows for more consistent and reliable monitoring of EEG signals and can be worn without being noticeable, which enhances both functionality and patient comfort,” said Tao Zhou, a professor of engineering science and mechanics at Penn State and the study’s co-corresponding author.
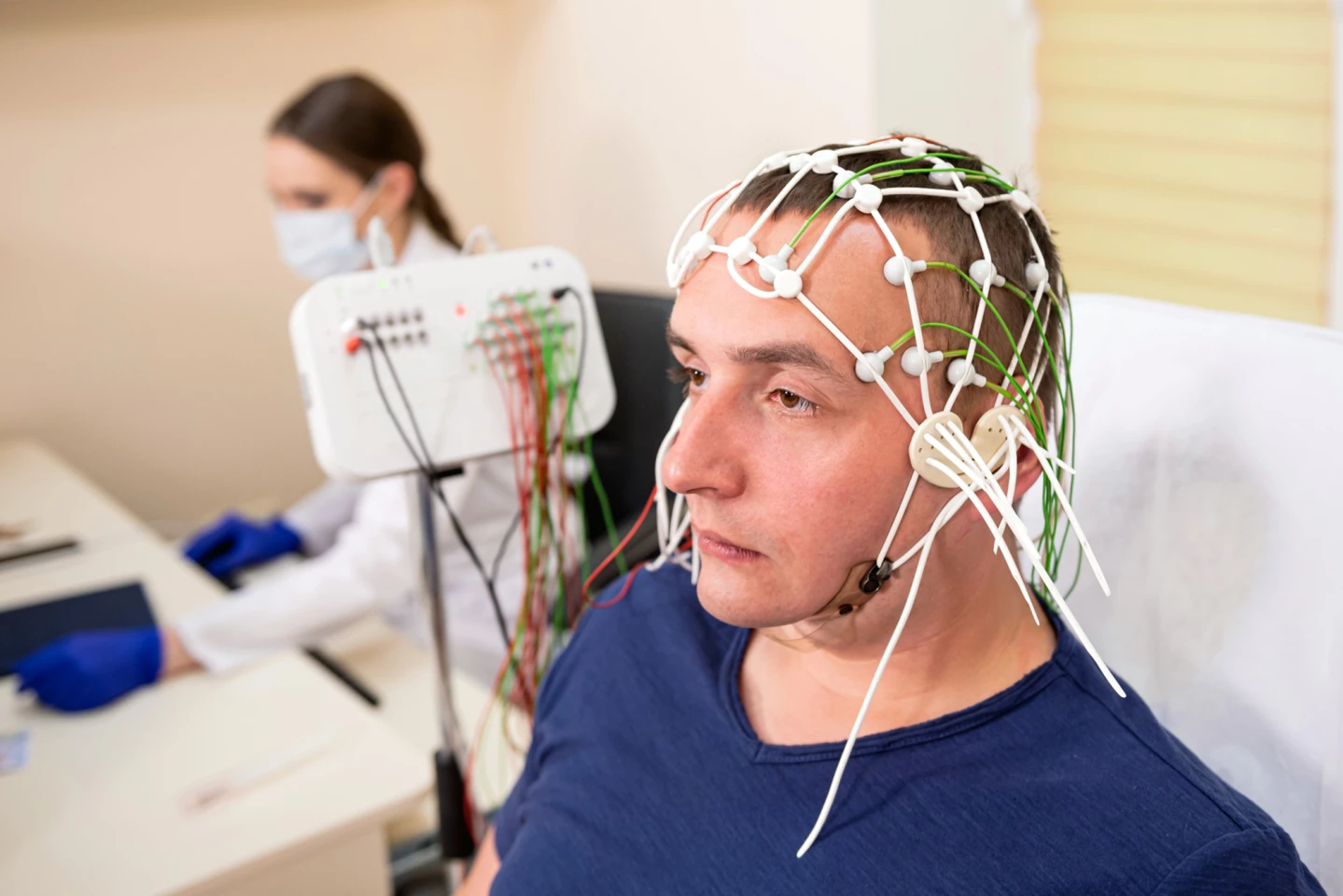
Used to record the brain’s electrical activity, an EEG is important for diagnosing and monitoring neurological conditions such as epilepsy, seizures, sleep disorders, and brain damage. The rigidity of the electrodes used in current EEGs means that even slight movement introduces noise and artifacts into the recording and limits the ability of patients to move freely. Commonly, “wet electrodes” are used, which require the application of conductive gel to maintain proper contact with the scalp. That approach is messy (think gel in the hair), and because the gel tends to dry out over time, it must be reapplied to maintain signal quality.
“This will change the impedance – or interface – between the electrodes and the scalp and it can affect the brain signal that’s recorded,” Zhou said. “We also don’t always apply the electrodes in the exact same position either because we’re human. But if you change the position, even a little bit, the brain signals you’re monitoring can be different.”
With these downsides in mind, Penn State researchers developed their gel-less “stick-and-play” device for EEG monitoring. It’s designed to be worn continuously for long periods while not interfering with the wearer’s usual activities or drawing attention to its presence. To that end, the electrode portion of the device is 3D-printed using a polymer hydrogel to be 300 μm wide. It looks like a single strand of hair. (By the way, it can be printed using different biocompatible dyes to ensure the device matches the hair color of the wearer.) The electrode is attached to the scalp using a 3D-printable bioadhesive that, on testing, was found to be almost double the strength of commercial EEG gel. It stayed on after showering and exercise-induced sweating, but did not cause damage to the skin when it was removed.
The researchers tested the device’s long-term adhesion and electrical performance and compared it to the current, standard EEG using multiple electrodes. Even while human test subjects performed their regular activities, the electrode remained securely attached to the scalp for 24 hours. Impedance remained stable with no noticeable increase after 12 or 24 hours, meaning that the quality of the brain activity signal didn’t change. The hairlike device maintained better skin contact than the regular gel-dependent approach and eliminated motion artifacts.
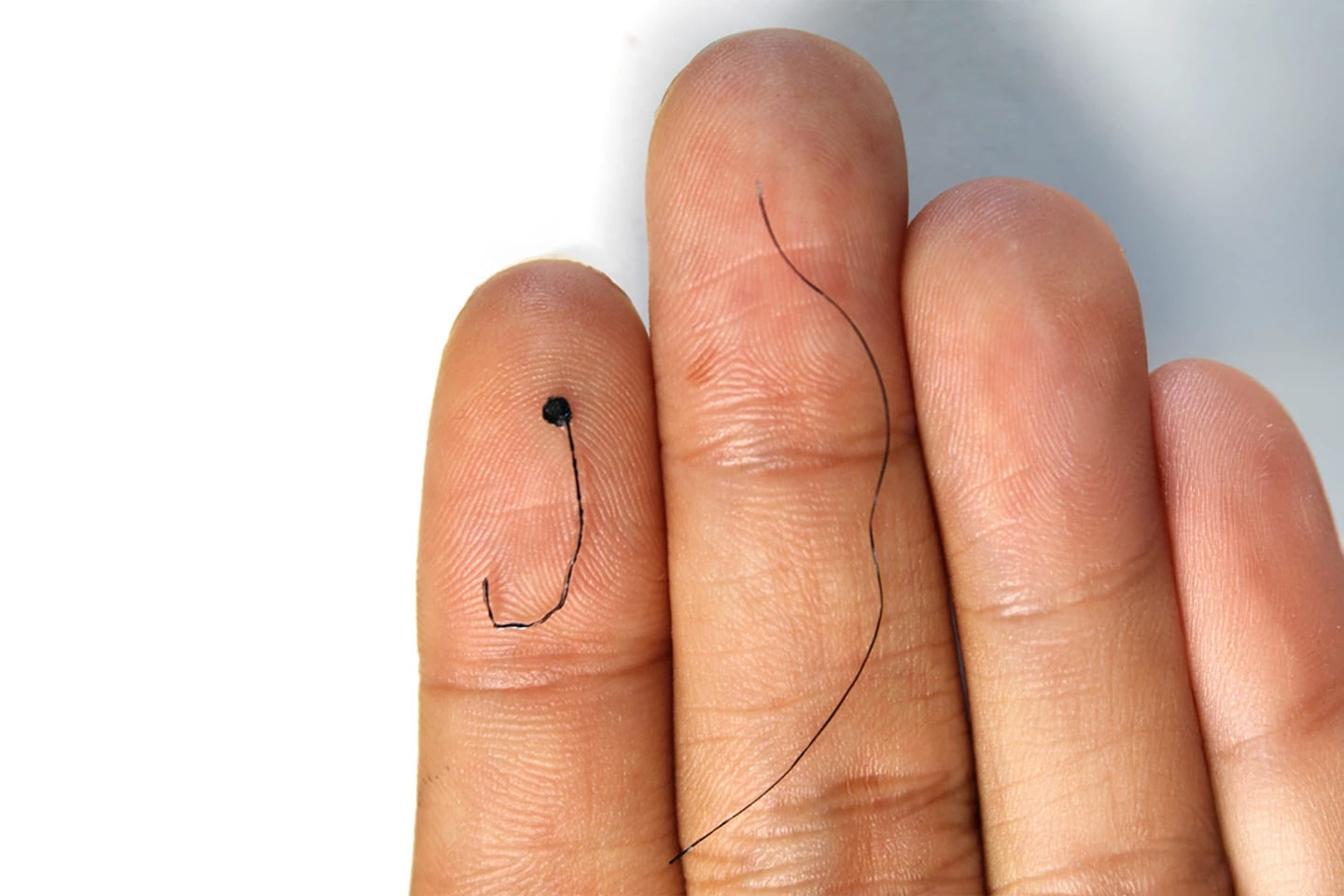
“You don’t have to worry if the position of the electrode has changed or if the impedance has changed because the electrodes haven’t moved,” said Zhou.
Currently, the hairlike EEG device is wired, so patients must be connected to a machine while their brain activity is recorded. However, the researchers hope to develop a wireless version and foresee many applications for the device.
“This technology holds promise for use in consumer health and wellness products, enabling the development of advanced wearable devices that can monitor mental health, stress levels, and cognitive functions discreetly,” they said. “It could also be integrated into brain-computer interface (BCI) systems, enhancing their usability and comfort, thereby expanding their application in fields such as assistive technology for individuals with disabilities, virtual reality (VR) experiences, and even enhancing human-computer interaction in everyday tasks.”
The study was published in the journal NPJ – Biomedical Innovations.
Source: Penn State