Researchers working at the Massachusetts Institute of Technology (MIT) claim to have created a method to better observe fermions – the sub-atomic building blocks of matter – by constructing a microscope capable of viewing them in groups of a thousand at a time. A laser technique is used to herd the fermions into a viewing area and then freeze them in place so all of the captured particles can be imaged simultaneously.
In the entire known universe, there are only two types of particles: fermions and bosons. In simple terms, fermions are all the particles that make up matter (for example, electrons), and bosons are all the particles that carry force (for example, photons).
Fermions include electrons, neutrons, quarks, protons, and atoms consisting of an odd number of any or all of these elementary particles. However, due to the strange (and not completely understood) nature of these particles in regard to their quantum spin states, scientists often opt to employ gases of ultra-cold fermionic atoms as proxies for other fermions.
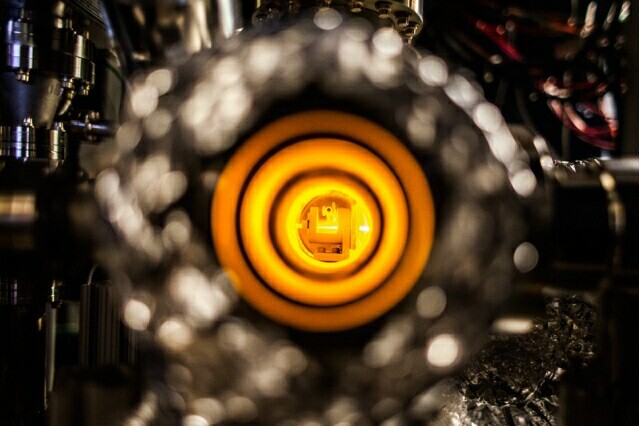
Over the last two decades, physicists studying ultracold atomic gases of boson particles – such as photons– have been able to do so relatively easily because bosons can occupy the same quantum state in boundless numbers. Fermions, however, are much harder to manipulate for imaging, as they are unable to be held in the same quantum state in large numbers and are very much more difficult to reduce to the temperatures required to slow them down enough to view them.
Physicists at HarvardUniversity successfully created a boson microscope that could resolve individual bosons in an optical lattice as far back as 2009. Similarly, in 2010, the MaxPlanck Institute of Quantum Optics also developed a second boson microscope.And, though these microscopes exposed the behavior of bosons, their counterparts – fermions – remained elusive without an equivalent fermion microscope. .
"We wanted to do what these groups had done for bosons, but for fermions," said Zwierlein says. "And it turned out it was much harder for fermions, because the atoms we use are not so easily cooled. So we had to find a new way to cool them while looking at them."
What is required to study fermions is a way to reduce their temperature, and therefore their movement, to a point low enough to image them. However, even techniques that resulted in the first ever laboratory realization of Bose-Einstein condensation in 1995 (which resulted in a Nobel Prize in 2001), or later work that saw lasers cool atoms to a few ten-thousandths of a degree above absolute zero are insufficient to achieve the cooling required to image fermion atoms.
To overcome this problem, the MIT researchers initially created an optical lattice using laser beams to form an arrangement of light "wells" which could magnetically trap and hold a single fermion in place (a technique similar to that used by the University of California to capture cesium atoms and image rotons). Applying a number of stages of laser temperature reduction, and more evaporative cooling of the gas (in this case, potassium gas), the atoms were cooled to just above absolute zero which was cold enough to hold individual fermions in place on the optical lattice.
As the fermions move to this lower energy state, they also release photons of light which can then be captured by the microscope and used to locate a fermion’s exact position within the lattice at an accuracy level greater than the wavelength of light.
"That means I know where they are, and I can maybe move them around with a little tweezer to any location, and arrange them in any pattern I’d like," said Martin Zwierlein, a professor of physics at MIT and a member of the team working on the project.
Unfortunately, this stability was tenuous because – when light was shone upon the atoms to view them – individual photons were able to knock them out of place.
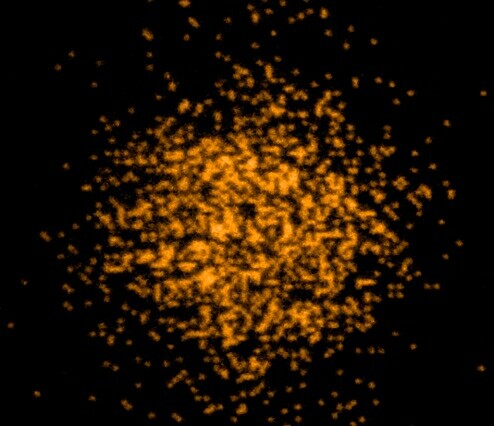
The team resolved this by cleverly employing a two laser beam approach where beams of differing frequencies were used to alter the fermion atom’s energy state. By simultaneously firing the two beams at the atom so that one beam frequency was absorbed by the particle, it would emit a corresponding photon in response. This, in turn, forced the particle into a lower energy state, thus cooling it further by reducing its excitation levels.
The upshot of this research, according to the team, is that the high-resolution image capture of more than1,000 fermionic atoms all together at the one time will help improve our fundamental understanding of these elusive particles. As electrons are also fermions, it is hoped that this information may eventually aid research into high-temperature superconductors, with their inherent advantages of lossless energy transport and the development of quantum computer systems.
"The Fermi gas microscope, together with the ability to position atoms at will, might be an important step toward the realization of a quantum computer based on fermions," said Zwierlein. "One would thus harness the power of the very same intricate quantum rules that so far hamper our understanding of electronic systems."
The results of this research have recently been published in the journal PhysicalReview Letters
Source: MIT