Researchers at the University of Pennsylvania have found a way to harvest energy from sunlight more efficiently, with the help of so-called plasmonic nanostructures. The new findings suggest that plasmonic components can enhance and direct optical scattering, creating a mechanism that is more efficient than the photoexcitation that drives solar cells. The development could therefore provide a real boost to solar cell efficiency and lead to faster optical communication.
When photons hit the surface of a solar cell, the energy they carry is absorbed by the atoms of a doped semiconductor. If the energy absorbed is higher than a set threshold, known as the energy gap, then electrons are set free and can be used to generate electricity.
Theoretically, the energy gap can be manipulated to maximize the number of electrons that will be set free by a photon; but setting this threshold isn't straightforward, because some photons carry more energy than others.
Photons in the infrared typically don't carry enough energy to knock electrons off a silicon atom. Red photons carry just enough energy to knock down a single electron, and photons in the blue spectrum and beyond carry enough to knock off one electron, but the rest of the energy is wasted as heat. This large amount of wasted energy compromises solar cell efficiency.
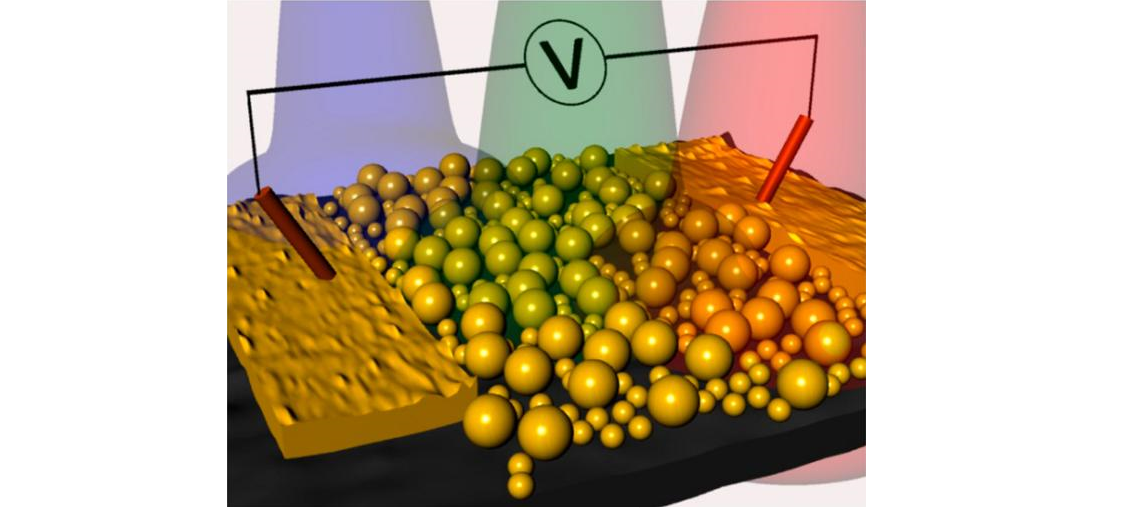
Building on their previous work, Prof. Dawn Bonnell and colleagues have now demonstrated that there is another way to harvest energy from light – a method that has tested up to 10 times more efficient than conventional photoexcitation and that could greatly improve the efficiency of solar cells and optoelectronic devices that convert light signals into electricity.
The University of Pennsylvania researchers focused on plasmonic nanostructures, materials made from arrays of gold nanoparticles and light-sensitive molecules of porphyin arranged in specific patterns.
When a photon hits these structures, it generates an electrical current that moves in a direction controlled by the size and the layout of the gold particles. By controlling and enhancing the way light scatters across them, these nanostructures can transduce light into electricity more efficiently than was previously possible. The freed up electrons can then be extracted from the plasmons and used to power molecular electronic optoelectronic devices.
Since their first results in 2010, the researchers led by Prof. Bonnell had suspected that their method could lead to significant increases in performance, but they couldn't prove it. Now, in this new study, they managed to do just that.
"In our measurements, compared to conventional photoexcitation, we saw increases of three to 10 times in the efficiency of our process," Bonnell says. "And we didn’t even optimize the system. In principle you can envision huge increases in efficiency."
"Light impinges on an array of metal nanoparticles connected with optically active molecules, and the resulting current is detected across the array," Prof. Bonnell tells Gizmag. "This process can produce more electrons in principle. You can imagine building energy harvesting devices made of the nanoparticles and organic molecules, or you could envision putting the nanoparticles into a silicon solar cell."
The nanostructures can be optimized for specific applications by changing the size and spacing of the nanoparticles, which would alter the wavelength of light to which the plasmon responds, in the same way that multi-junction solar cells are built to absorb photons of different wavelengths more effectively.
Applications could include the more efficient transduction of optical signals (e.g. from fiber optics) into electrical signals and, of course, more efficient solar cell technology. "You could imagine having a paint on your laptop that acted like a solar cell to power it using only sunlight," Bonnell says.
Promising as they may sound, we must remember that these results are largely theoretical; through this study the researchers have shown that generating electricity using plasmonic nanostructures can be more efficient than by using standard photoexcitation, but there's no telling how soon devices exploiting this principle could reach mass-production, or even what kind of actual efficiency gains they could bring.
The results were recently published on the journal ACS Nano.
Source: University of Pennsylvania