If you looked inside any cell in your body right now, you'd notice that the individual structures and components within it are constantly moving around. While some of that twitching and jostling is passive, other movement is more deliberate, with the cell actively exerting energy to move components. A new data analysis technique is improving our ability to distinguish between those two types of movement, and the results could significantly improve our understanding of cell biology.
When you zoom down to the microscopic scale, particles within fluids or gases are easily influenced by their surroundings. We first learned this back in 1827, when Scottish botanist Robert Brown studied pollen grains in water through a microscope, and noticed that the grains contained tiny particles that moved continuously.
We now know that the jiggling of the cell's interior particles is due to their interaction with water molecules, which, at elevated temperatures, possess perpetual kinetic energy. The particles continuously bombard larger components inside cells, causing seemingly random movement. Thanks to its discoverer, we call this Brownian motion.
The phenomenon is so common that scientists routinely dismiss in-cell movement as occurring at thermal equilibrium, with the system not exerting energy to move the component, which if it wasn't for the Brownian motion, would be inanimate.
However, this isn't, of course, always the case – sometimes a cell is exerting energy to move a component around. The problem is – how do we work out when one or the other type of motion is occurring?
That's where the new analytical method steps in. Developed by researchers at MIT, in collaboration with scientists at the University of Gottingen, the University of Munich, Free University Amsterdam and Yale University, it's designed to pick out those times when the movement is active rather than passive, simply by looking at a particle.
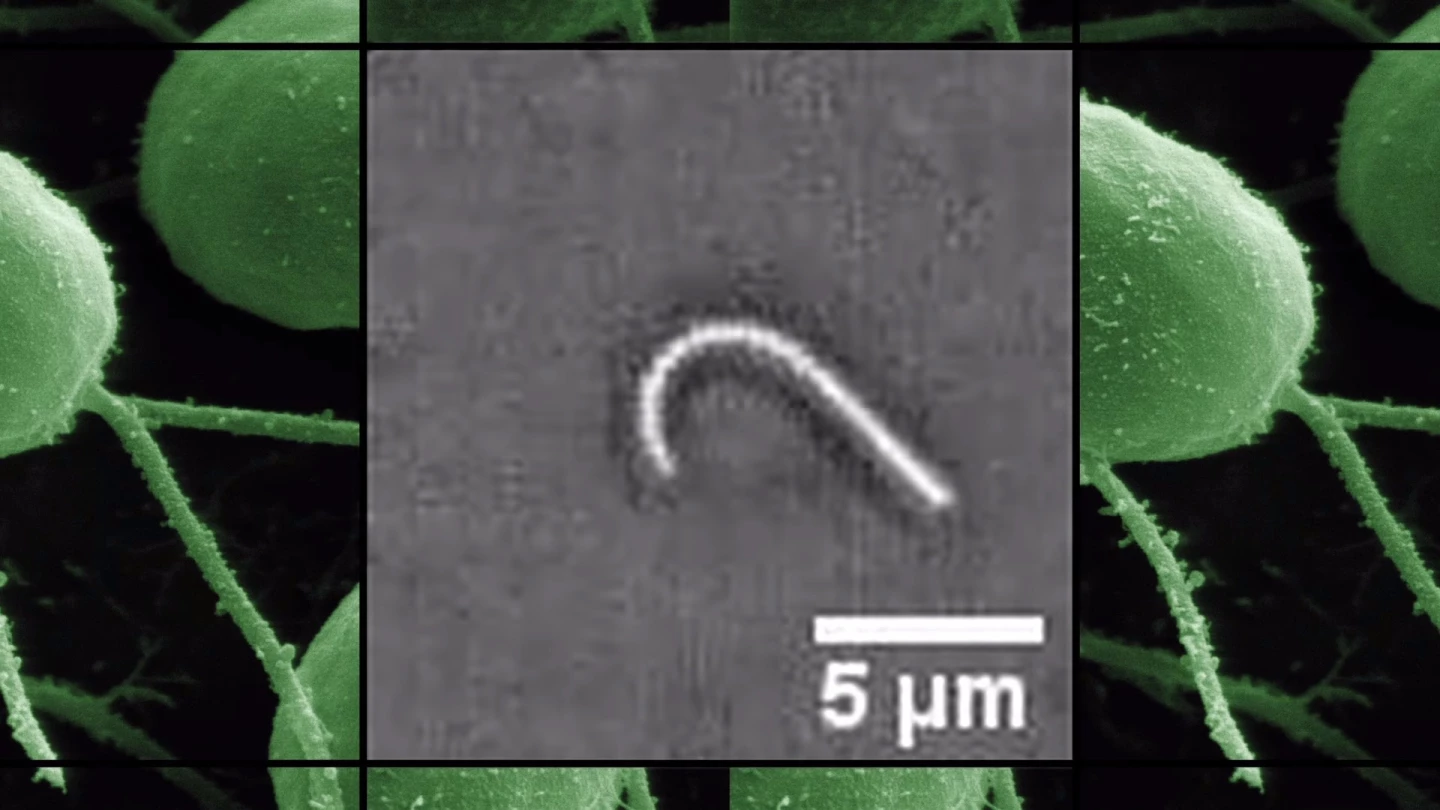
The team used video microscopy to study the motion of flagellum frame-by-frame, looking carefully at the changes in its backbone and identifying the different shapes it formed as it passed through a complete oscillatory cycle. If the flagellum was in thermal equilibrium (passive), then back-and-forth transitions between states should be balanced, but instead observed an imbalance in the transitions, suggesting that energy is actively being expended – something that's already confirmed in the case of flagellum.
Knowing that the method worked, the researchers then conducted the same experiment with a kidney cell cilium, where they once again observed a slight imbalance in transitions. The results in that case were more surprising, indicating that active processes drive the movement of the cilium, in contrary to its passive appearance.
The research is potentially revolutionary, providing an unparalleled insight into how cells function, uncovering active processes that are far from obvious at first glance.
"We want to see if particular dynamics in livings systems – be those cells or tissues or whole organisms – that look at first glance like random thermal motion are indeed actively driven," said paper co-author Professor Nikta Fakhri. "This is important because there must be a vital function connected with the process if the cell spends energy on it."
Full details of the research are published online in the journal Science. For more on the study, you can take a look at the video below.
Source: MIT