Gravity waves are the big ticket item of physics. Predicted by Albert Einstein in 1916 as part of his general theory of relativity, these waves could help scientists solve many mysteries about the origin of the universe – if they could detect them. In an attempt to do this, researchers at Stanford University and NASA’s Goddard Space Flight Center are developing a new atomic interferometry technique that may be sensitive enough to record gravity waves for the first time.
Gravity waves are ripples in the space-time continuum caused by catastrophic events like colliding supermassive stars or the Big Bang. When they’re generated, it’s as if the universe was ringing like a gong. If they could be studied, these waves could provide scientists with a treasure trove of information on everything from black holes to being able to see back to the earliest moments of the Big Bang.
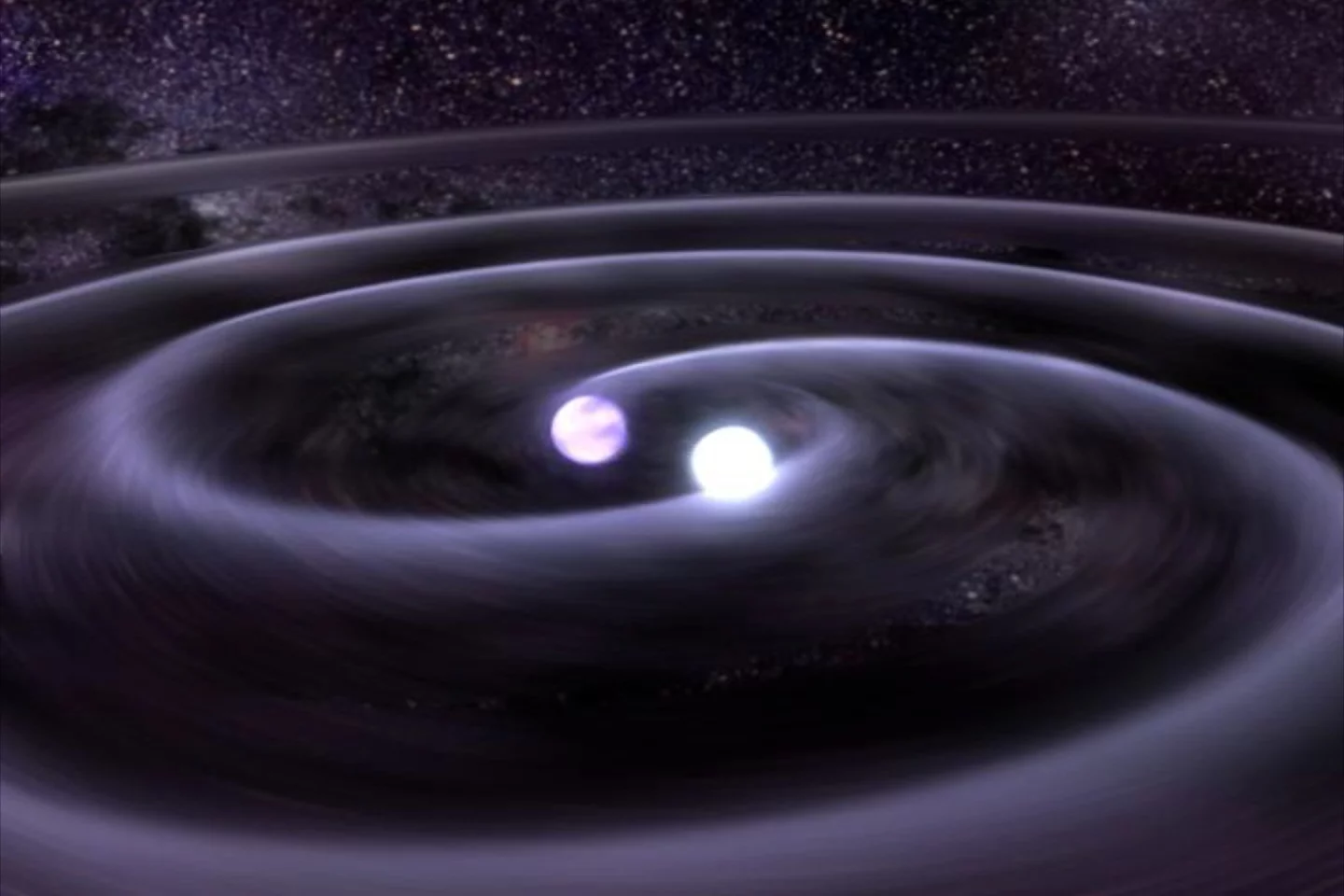
The problem for scientists is that these waves are very faint and when the Earth passes through one it expands and contracts by less than the width of an atom. Not surprisingly, this incredibly tiny response is extremely difficult to detect on even the most sensitive instruments. What the Stanford/Goddard team are trying to do in conjunction with AOSense, Inc. of Sunnyvale, California is to develop an interferometer that uses atoms instead of light to produce an ultrasensitive detector.
An interferometer is a device that can detect very small changes with great accuracy. The most familiar one from classroom laboratories uses light, though radio waves, x-rays and just about any other waves can be used. The basic principle is that a light ray is passed through a beam splitter and broken into two new beams. One beam travels along the original path and the other is sent off at a right angle. These beams are bounced off of mirrors and sent back the way they came and then the beam splitter directs both into a detector. The waves that make up the two beams then interfere with one another, forming a pattern.
The clever bit in all of this is that if something alters one of the beams, such as the distance traveled changing by the tiniest fraction, this changes the resulting pattern and from that scientists can deduce how big the change was. The sensitivity of the instrument depends on the wavelength used, the distance of the paths traveled and the stability of the instrument.
In the case of atomic interferometry, the Stanford/Goddard team is exploiting an oddity of quantum mechanics that states that if an atom is cooled to near absolute zero it takes on the properties of a wave. According to Mark Kasevich, a Stanford University professor and team member, "Atoms have a way of being in two places at once, making it analogous to light interferometry."
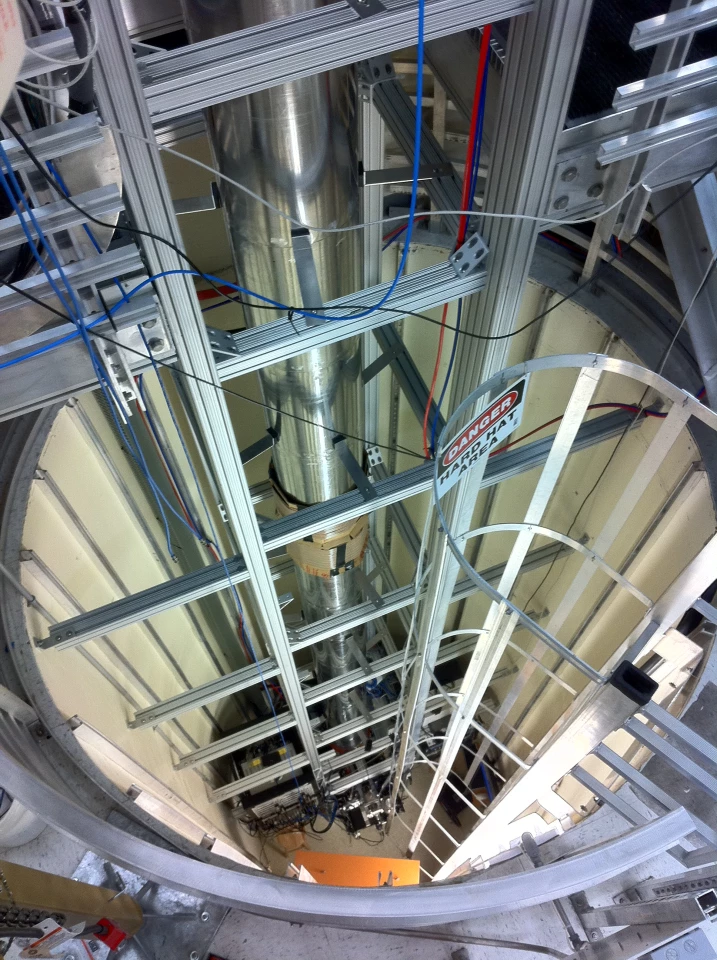
What the team will do is insert a cloud of neutral rubidium atoms inside the 33-foot (10 m) drop tower in the basement of a Stanford University physics laboratory. As these atoms fall, they’ll be zapped with a laser to cool them down and spread them out. Because of the peculiarities of quantum mechanics, the rubidium atoms act like a light interferometer turned inside out with the lasers that make up the detector acting as the beam splitter and mirrors while the atoms give off “matter waves” due to a “superposition of states.”
The upshot of all of this is an interferometer that can detect a change of a picometer – that’s one trillionth of a meter. To put that into perspective, a helium atom is 62 picometers across.
The team hopes that this device will not only be sensitive enough to detect gravity waves, but that it will also be used for ultra-precise navigation for aircraft, submarines and asteroid-exploring probes. If the technique proves successful, the team plans to adapt it for a space mission consisting of three probes flying in a triangular formation 500 to 5,000 kilometers (310 to 3,100 mi) to form a gigantic atomic interferometer of even greater sensitivity.
Source: NASA