Hydrogen transport and generation powder Si+ was revealed last week, causing quite a stir with its promise of making renewable energy incredibly cheap, convenient and safe to transport – in stark contrast to the expense, inefficiencies and difficulties involved in transporting hydrogen as a gas, a cryogenic liquid or embedded in ammonia.
Much of the world seems to be coalescing around green hydrogen as a clean way to store, transport and export renewable energy, but as we outlined in another piece last week about a hydrogen storage powder developed by scientists at Australia's Deakin University, the high costs involved in compressing and containing the gas, cryogenically cooling and storing the liquid, or converting it to ammonia could place a heavy thumb on the cost scales. The green energy transition can't happen if the costs are so high that economies can't bear their weight.
Enter the solid-state hydrogen powders. Whether it's Deakin's ball-milled boron nitride or EPRO Advance technology (EAT)'s porous silicon, these powders can effectively transport green energy using cheaper and much more conventional means. Fill a truck or a shipping container with either, send it on a regular cargo boat, and somebody at the other end can use it to release hydrogen, ready to go.
Deakin's solution requires heat for the hydrogen release, EAT's Si+ product requires water and sodium hydroxide. Both offer a zero-emissions pathway, and leave the user with by-products that are either recyclable or potentially useful. And both claim to be radically cheaper than pure hydrogen, particularly when transport and storage costs are factored in.
EAT's Si+ powder is claimed to offer a higher density of energy storage per weight than the Deakin powder, and once combined with alkaline water to produce its hydrogen, it leaves nothing behind but silica, which can be sold off to make concrete. So it's of particular interest.
Naturally, you had questions. We did too, so to follow up from our original piece, we contacted EAT Executive Director and CEO Albert Lau over email. We put the following questions to him, and present Lau's answers below, in an edited format.
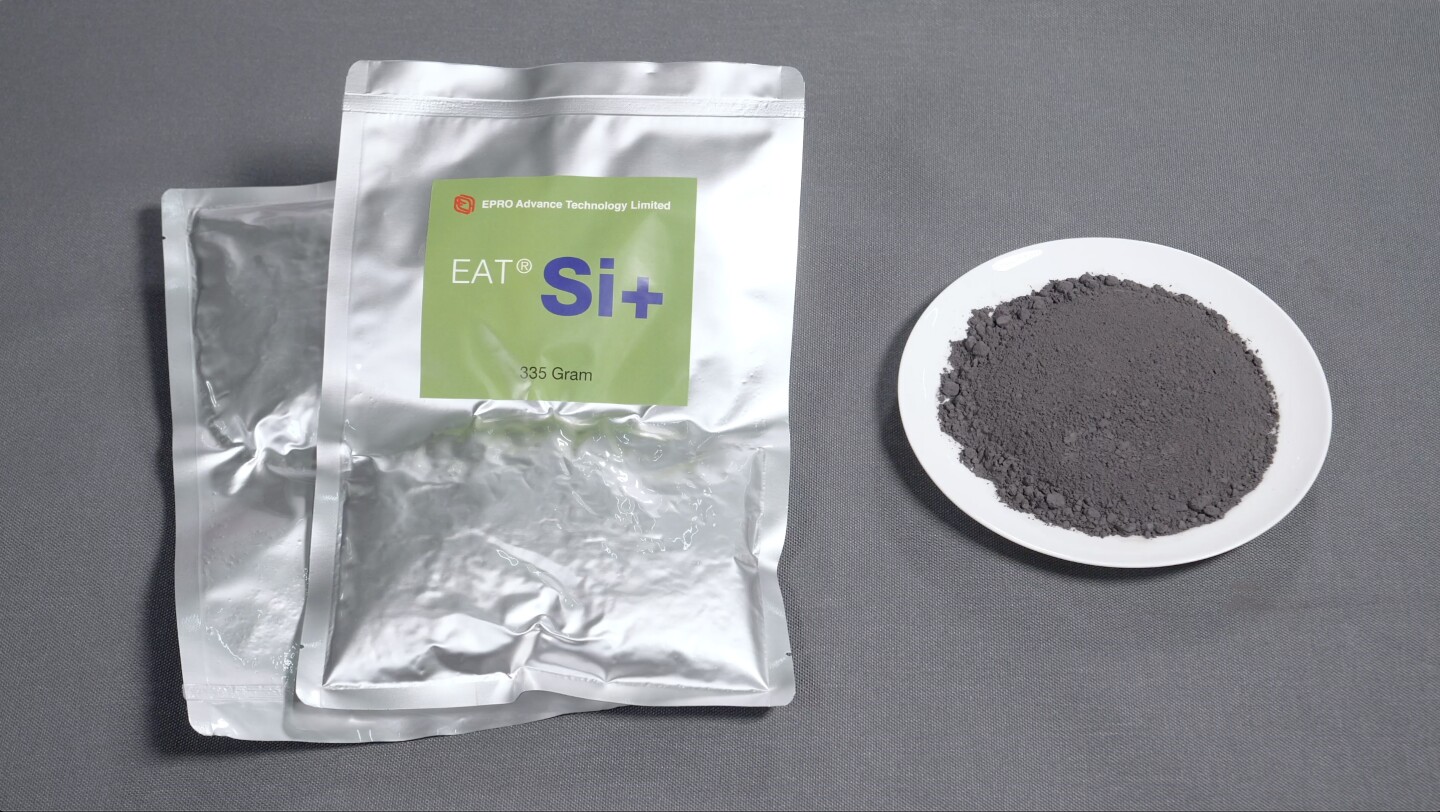
1) What's the energy efficiency of creating the Si+ powder? How much energy goes in compared to what's released?
Round trip efficiency of Standard Si+ (using grid electricity starting from sand/quartz with a carbon source) amounts to around 48%. This includes the exothermic heat that is exerted during the hydrogen generation process as well. Forty-eight percent is lower than what electrolyzer systems give, but when there is transportation and storage required, Si+ wins.
2) Do you have a sense of what it'll cost, compared to gaseous hydrogen? Both in terms of the material itself and perhaps in an export shipping scenario?
Cost will depend heavily on where the hydrogen is produced and where it has to be transported to be used. Usually renewable energy resources are remote, which calls for transportation.
To provide some perspective on delivery cost of H2, the Kawasaki Heavy Industries liquid hydrogen ship costs US$362 million, and a round trip is expected to take around 60 days. The calculations are straightforward: let’s use 30 years depreciation, a $500 per ton scrap value on this 8,818-ton vessel, 60 days as the length of each round-trip shipment, and let’s assume there's zero boil-off during the 60 days of shipment, such that the ship actually delivers a full 88.5 T of hydrogen. Simple calculations will give us a depreciation cost alone of ~ 22.1 US$/kg H2, under the most generous assumptions, and not including any operating costs of the ship and/or profits to the companies involved, nor any land-based costs either!
Australia and Japan aren’t the only countries progressing with liquid hydrogen. Shell New Energies NL, ENGIE, Vopak and Anthony Veder have signed an agreement to study the feasibility of producing, liquifying and transporting green hydrogen from Portugal to the Netherlands as well!
3) Would the Si+ powder react with moisture in the air? If so, how must it be stored and handled?
Yes, Si+ will react with moisture in the air! However, without sodium hydroxide (NaOH), that reaction cannot be sustained. The Si+ will form a passive oxide layer on the surface, which protects the inner core of the Si+ pellet from further reactions. Also, without the basic conditions, hydrogen generation reaction is extremely slow.
Si+ will be vacuum packed, or in some cases, purged with an inert gas such as nitrogen or argon.
4) How much water does it require? Say you're carrying 7.4 kg (16.3 lb) of Si+ powder to release 1 kg (2.2 lb) of hydrogen, how much water do you need to add? And how pure does the water need to be?
This is a complex question of chemistry and molecular dynamics. To simplify the response, 30 L of water can react with 7.4 kg of Si+ over the course of several hours. Water can be filtered surface water.
5) What sort of equipment and material inputs are needed to manufacture the Si+ powder? Is this something that could be easily rolled out at wind farms and solar arrays for instant downtime generation?
Si+ is produced via metallurgical means, meaning large foundry furnaces that operates at high temperatures. An Si+ foundry can definitely be set up to cope with these curtailed and under-utilized renewable energies!
6) How much heat does the release reaction release? Can it be managed or used?
Around 24 kWh of low grade heat (of up to 70 °C/158 °F) will be generated per kg of H2 generated. This heat energy can be recovered by heat exchangers, which can then be used for space heating, domestic or commercial water heating, etc. In some extreme cases, this heat can be converted back into electricity via thermo-electrics.
We thank Mr. Lau for his enlightening responses. Certainly, the weight of the water required and the heat energy released will be important considerations in how Si+ might be used. Moisture will clearly be a factor in transport, meaning that a lot of this powder may end up being packed in plastic.
As far as the efficiency, well, NEL Hydrogen claims its A series electrolyzer can produce a normal cubic meter (35.3 cu ft) of hydrogen (storing roughly 3 kWh of usable energy) using just 3.8 kWh of energy, representing an efficiency somewhere around 79% – but this obviously doesn't factor in the considerable energy costs associated with compressing that gas to 700-odd atmospheres or cooling it to a cryogenic 20 Kelvin (-253 °C / -424 °F) for storage and transport. So it may well come out significantly more efficient in the wash.
We look forward to seeing how these hydrogen powder innovations play out on the commercial stage.
Check out the Si+ launch video below.
Source: EAT Si+