The phrase "engage the ion drive" still has the ring of a line from Star Wars, but these engines have been used in space missions for more than four decades and remain the subject of ongoing research. Ion engines have incredible fuel efficiency, but their low thrust requires very long operating times ... and therein lies the rub. To date, erosion within such an engine seriously limits its operational lifetime. Now a group of researchers at NASA's Jet Propulsion Laboratory (JPL) has developed a new design that largely eliminates this erosion, opening the gates for higher thrust and more efficient drives for manned and unmanned missions to the reaches of the Solar System.
Ion engines of various types have been used on space missions since at least 1964, when NASA flew the suborbital Space Electric Rocket Test I mission. Many classes of space missions can benefit through using fuel efficient ion engines during some phase of their mission. For example, several communication satellites have been raised into their final geosynchronous orbit using ion thrusters. The European Space Agency's SMART-1 lunar mission was placed in geosynchronous orbit by conventional means, and then made the transfer into lunar orbit using an ion engine.
Deep space missions, however, is where ion engines could really shine. Three missions, NASA's Deep Space One and Dawn, and the Japan Aerospace Exploration Agency's Hayabusa have partially or entirely obtained their post-Earth-orbit propulsion from ion engines. Their ion engines operated for several years with only an occasional panic attack while providing a few hundredths of a Newton (perhaps 0.4 oz) of thrust.
How does an ion engine work?
There are many varieties and more proposals (the VASMIR engine comes to mind), but the operating principle is quite simple. There are two basic styles of ion engines, electrostatic and electromagnetic. An electrostatic ion engine works by ionizing a fuel (often xenon or argon gas) by knocking off an electron to make a positive ion. The positive ions then diffuse into a region between two charged grids that contain an electrostatic field. This accelerates the positive ions out of the engine and away from the spacecraft, thereby generating thrust. Finally, an neutralizer sprays electrons into the exhaust plume at a rate that keeps the spacecraft electrically neutral.
An electromagnetic ion engine also works by ionizing a fuel. In this case a plasma is created that carries current between the ionizing anode and a cathode. The current in turn generates a magnetic field at right angles to the electric field, and thereby accelerates the positive ions out of the engine via the Lorentz force – basically the same effect on which railguns are based. Again a neutralizer keeps the spacecraft electrically neutral.
Powering a serious spacecraft
This all takes a good amount of electrical power – about 25 kW per Newton (3.6 oz) of thrust. So what thrust levels are needed to push, say, a 100 ton spacecraft throughout the solar system? (Forgive me – I like to dream!) It depends on the mission, of course, but 1000 N of thrust would put that spacecraft in orbit around Jupiter in about 10 months and in Neptunian orbit in just under 1.5 years. Obviously this is pretty far down the pike technologically, but let's see what is needed.First, a supply of electrical power that delivers about 25 MWe (megawatts of electric power) more or less constantly. Clearly, we're talking about nuclear power – a lot of nuclear power from a reactor system that fits within a 100 ton spacecraft. Fortunately, there is currently a good deal of effort going into designing compact nuclear reactors for power production here on Earth.
In addition, NASA and DOE are collaborating on the Fission Surface Power Project, where the goal is to make tiny nuclear power reactors for bases on the Moon and Mars. The design goal is to produce a reactor that will provide 40 kWe for 10 years, fold into a 3 x 3 x 7 meter (10 x 10 x 23 feet) space and weigh in at 11,000 lbs (5000 kg). This is quite a ways from what is needed for a 1000 N ion drive, but with molten salt reactors and efficient conversion of heat into electricity, it seems within the bounds of possibility. Besides, I said I was dreaming.
Design roadblock
If the above were worked out satisfactorily, could we build a 1000 N thrust ion engine? There are some minor technical problems with efficiently ionizing the fuel and cooling the engines, but the biggest roadblock of which we are currently aware is that the large ionic current passing through the engine will cause enough erosion to destroy the engine. This is not a materials problem – it is a design issue. This is the roadblock recently demolished (at least partly) by NASA researchers at the Jet Propulsion Lab in Pasadena, California.
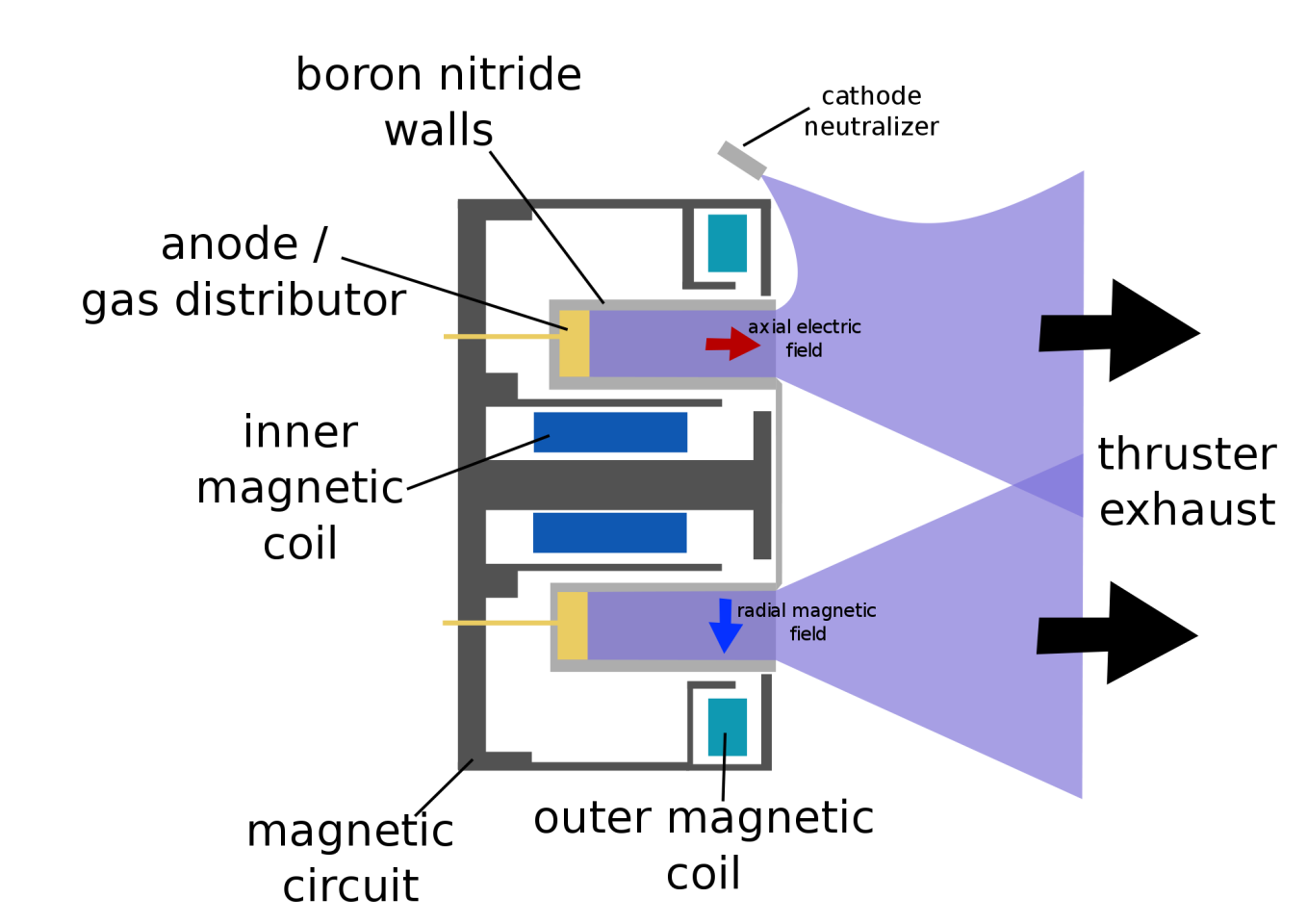
You can see in the cross-section diagram above that the fuel plasma fills the anode and gas distributor. At low thrust, the small plasma density is accelerated by the Lorentz effect of the crossed magnetic and electric fields. However, at large thrust, the plasma density becomes large enough to distort the fields, resulting in positive ions being accelerated directly into the anode walls.
When these ion energies are large enough, they will erode material from the walls in a process called sputtering. To make matters worse, in the quest for better ion engines, one desires both larger thrust and larger exhaust velocities (which require less fuel). Changes made to meet both of those goals greatly increase the rate of erosion.
This problem is made more difficult because the electrodynamics of the fields and the plasma are seriously nonlinear, making it difficult to predict the effect of a change in engine design on the erosion of the engine.
The obvious approach was to magnetically shield the walls from the energetic ions. The NASA team accomplished this by shielding the boron nitride walls so that the magnetic field from the inner and outer magnetic coil would pass around the end of the anode annulus. Properly done, the magnetic field no longer penetrated the walls. As a result, the magnetic field lines, rather than penetrating the walls at angles close to perpendicular, are nearly parallel to the walls. This causes the positive ions to be accelerated away from the walls, and as a result the walls are effectively the coolest part of the internal engine surfaces.
The result of experimental tests of the new magnetically shielded configuration showed the rate of erosion was reduced by a factor of 500-1000. This highly successful demonstration took place in a six kW Hall effect ion thruster.
While there will no doubt be more challenges to overcome as work proceeds in the further development of large-scale ion drives, this new research looks to have solved the nearest and most visible problem. Manned deep space missions are one step closer, and having dreamed of space travel all my life, I'd like to live to see it happen.
Sources: Applied Physics Letters, NASA-JPL