Scientists have struggled for decades to identify the constituent particles of dark matter, but they’ve had little to show for all their efforts. A new study at Case Western Reserve University is now advancing the radical new hypothesis that dark matter may in fact be made not of exotic subatomic particles, but rather of macroscopic objects which would mass anywhere from a tennis ball to a dwarf planet, be as dense as a neutron star, and still be adequately described by the Standard Model of particle physics.
Dark matter constitutes approximately 27 percent of the mass-energy of the Universe, trumping the measly five percent of ordinary matter. Although it can’t be observed directly, we know it must be there because of the strong gravitational effects it exerts on ordinary matter as it distorts incoming light and helps new galaxies form.
The search for the dark matter particle has been fruitless thus far and so, in an effort to leave no stone unturned, researchers Glenn Starkman and David Jacobs have chosen to take a look at the bigger picture, focusing on what the dimensional constraints of a dark matter particle actually are. Over the past 30 years, the search has mainly focused on the space of weakly-interacting particles such as WIMPs and axions. But in expanding their field of view, Starkman and Jacobs have concluded that the objects which interact strongly with both themselves and ordinary matter deserve more attention, not least because they could be accounted for by the currently accepted Standard Model of physics.
"The community had kind of turned away from the idea that dark matter could be made of normal-ish stuff in the late ‘80s," Starkman said. "We ask, was that completely correct and how do we know dark matter isn’t more ordinary stuff – stuff that could be made from quarks and electrons?".
When looking at the constraints in terms of mass and cross-section of such hypothetical objects, something immediately jumps to the eye: such objects could be absolutely massive, and as dense as a neutron star or the nucleus of an atom. Which explains the researchers’ nickname for them – the "Macros."
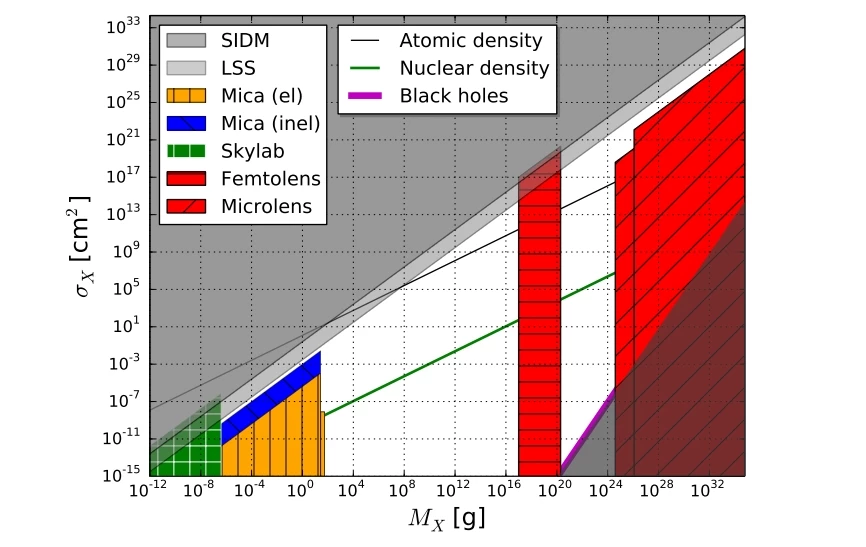
The white areas in the graph above represent the ranges of mass and cross-sectional area under which such Macros could theoretically fall. The colored areas indicate ranges that must be excluded as they already characterize objects such as failed Jupiters, white dwarfs, neutron stars, stellar and supermassive black holes and neutrinos. For reference, the green line indicates the volumetric density inside the nucleus of an atom.
According to the team’s results, dark matter should mass somewhere between 55 grams (1.9 oz), which is as much as a tennis ball, and 1024 grams, which is around the mass of the dwarf planet Ceres.
The researchers say that the Macros couldn’t fall outside this interval because a smaller object would have already been detected with existing instruments, while a larger object would bend starlight to a degree that hasn’t been observed so far. Similarly, the Macros also cannot mass between 1017 and 1020 grams, as they would have affected individual photons coming from gamma ray bursts in ways that have not seen.
So, what would such massive objects be made of? Starkman says that matter with a considerable percentage of so-called strange quarks is a possible candidate. This type of quarks ordinarily live extremely short lives, but the researchers say there’s a possibility that stable strange nuclear matter, or perhaps other forms of baryonic matter, may have formed in the early Universe at temperatures above 3.5 trillion degrees Celsius (6.5 trillion degrees Fahrenheit), comparable to the temperatures in the center of a massive supernova.
"Dark matter [could be] nothing more than chunks of strange nuclear matter or other bound states of quarks, or of baryons, which are themselves made of quarks," says Starkman.
If dark matter is indeed macroscopic, there may be explanations as to why it hasn’t been discovered yet. If its mass is very large, it would only hit Earth once every billion years; conversely, at masses on the lower end of the spectrum, it would hit Earth very often, but without leaving a discernible mark.
However, the researchers say that if the Macros mass between 100 and 1017 grams, then there could be up to a million billion such objects inside the sphere defined by Earth’s orbit around the Sun. Careful observation of this region could then give us the answers to questions we've been asking for decades.
An open-access paper describing their work can be found on the ArXiv website.
Source: Case Western Reserve University