Arguably the world’s most famous painting, da Vinci's Mona Lisa has now been copied onto the world’s smallest canvas at the Georgia Institute of Technology. Associate Professor Jennifer Curtis' "Mini Lisa" is one-third the width of a human hair, with details as small as one-eighth of a micron. Mini Lisa demonstrates the flexibility of a new nanolithography technique that can vary the surface concentration of molecules on very small portions of a substrate.
Mini Lisa was rendered using an atomic force microscope and a new process called ThermoChemical NanoLithography (TCNL).
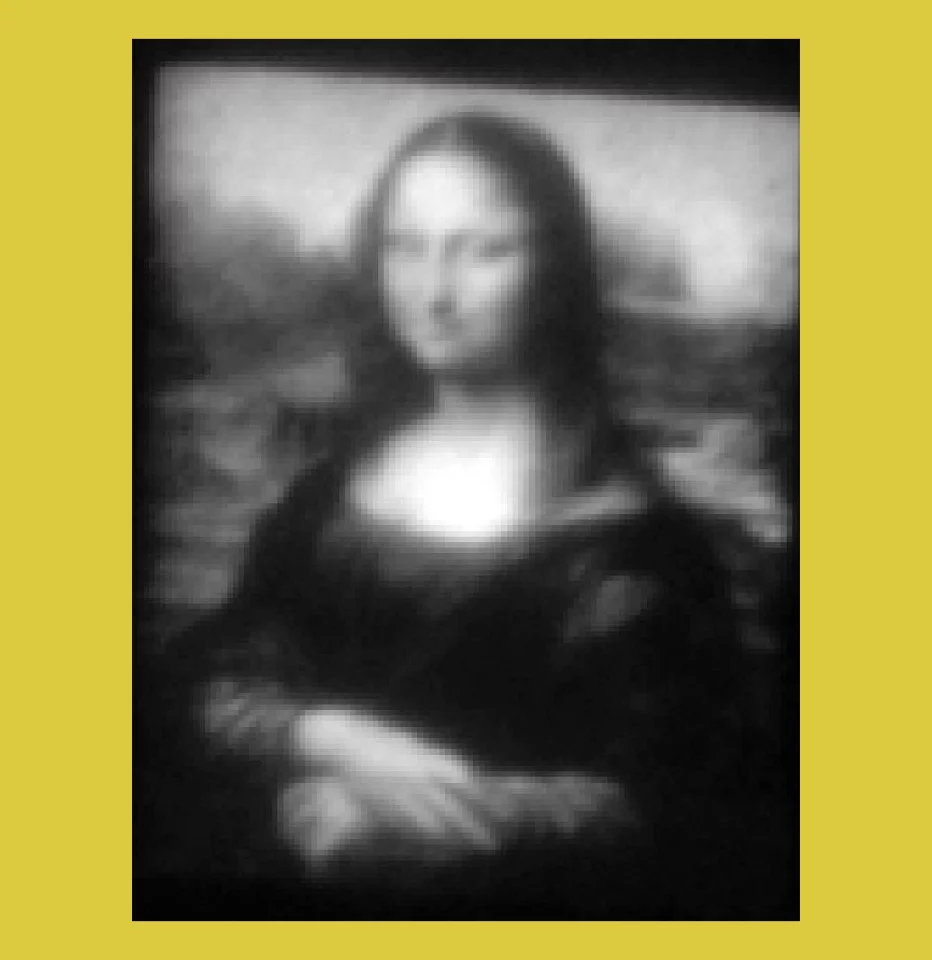
The Georgia Tech team formed the image pixel by pixel. The cantilever and tip of an atomic force microscope were heated, and then lowered to the desired location of a pixel.
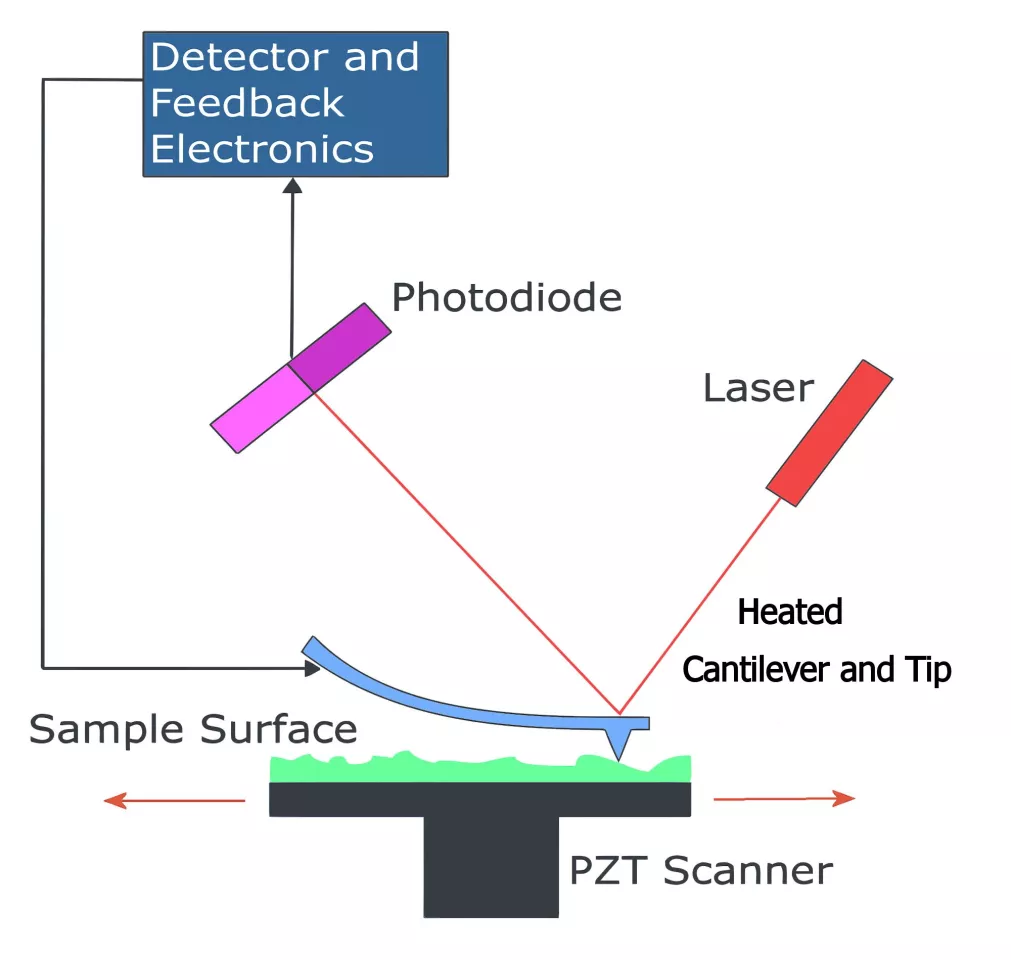
The molecular canvas is a plastic whose surface contains active chemical sites which are initially protected from chemical reaction by capping them with protecting molecules. The heat of the cantilever triggers a confined nanoscale chemical reaction, in which more heat produces a greater local concentration of new surface molecules.
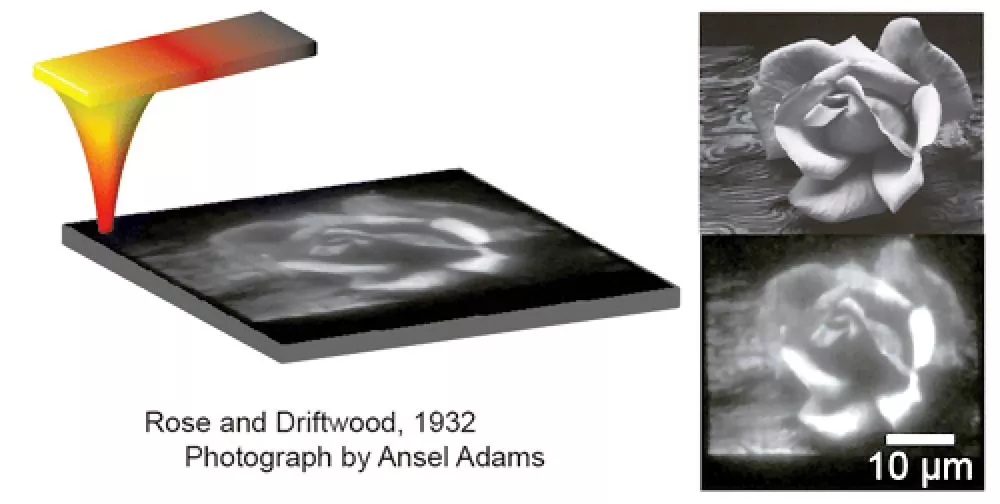
More heat produced the lighter shades of gray, as seen on the Mini Lisa’s forehead and hands. Less heat produced the darker shades in her dress and hair seen when the molecular canvas is visualized using fluorescent dye. Each pixel is spaced by 125 nanometers, and the overall image has 240 x 320 pixels.
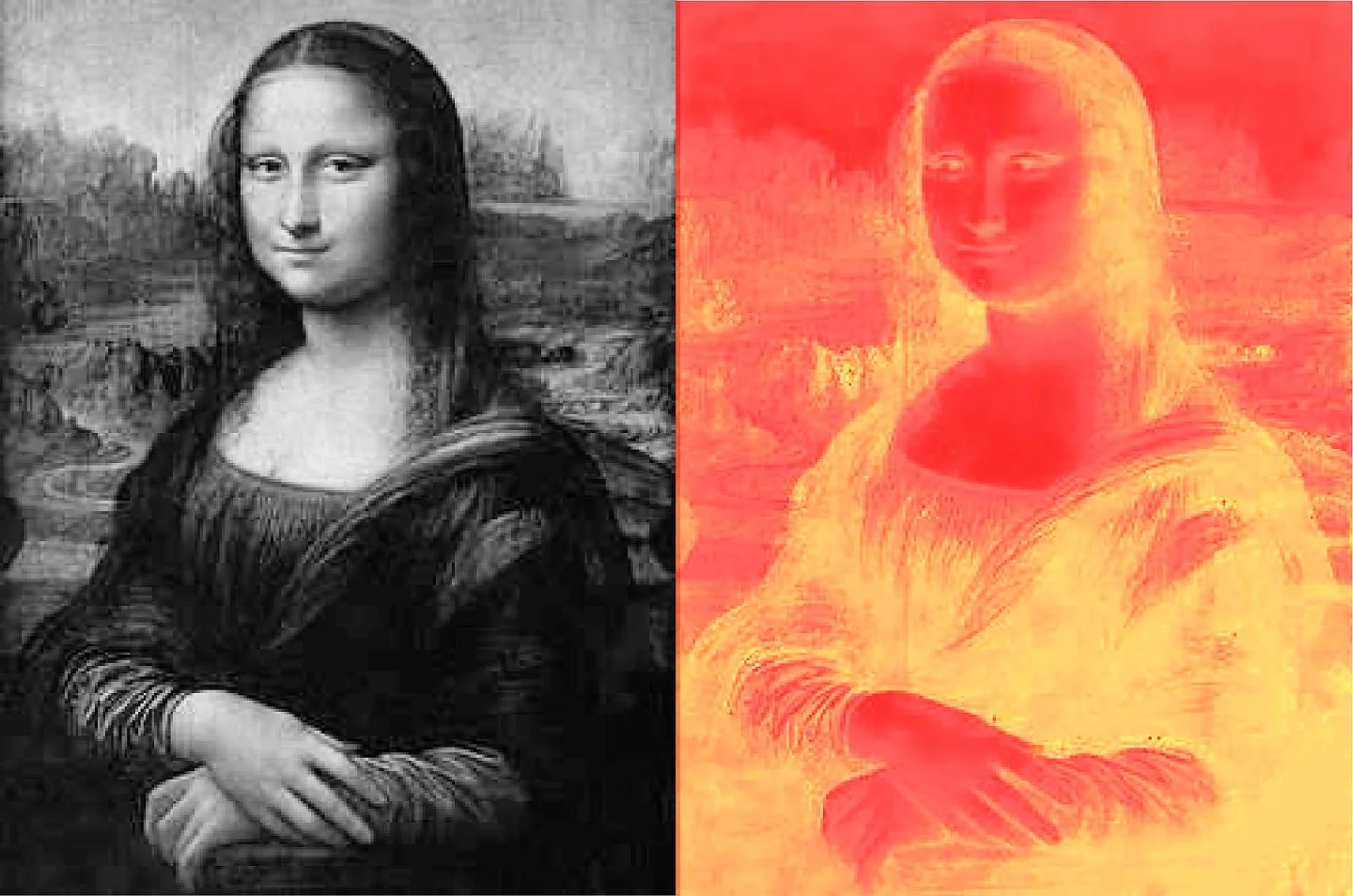
“By tuning the temperature, our team manipulated chemical reactions to yield variations in the molecular concentrations on the nanoscale,” said Jennifer Curtis, an associate professor in the School of Physics and the study’s lead author. “The spatial confinement of these reactions provides the precision required to generate complex chemical images like the Mini Lisa.”
Generating chemical concentration gradients and variations on the sub-micrometer scale is difficult to achieve with other methods. At present the Georgia Tech TCNL system is limited to production of chemical gradients of amine and carboxyl groups, but it is expected that other material chemistries will be compatible with the new process.
“We envision TCNL will be capable of patterning gradients of other physical or chemical properties, such as conductivity of graphene,” Curtis said. “This technique should enable a wide range of previously inaccessible experiments and applications in fields as diverse as nanoelectronics, optoelectronics and bioengineering.”
This simple technique renders high spatial resolutions at a speed faster than other existing methods. While one obvious area of application is in making specialized surfaces for "lab on a chip" development, Professor Curtis is hopeful that TCNL will provide the option of nanoscale printing integrated with the fabrication of large quantities of surfaces or everyday materials whose dimensions are more than one billion times larger than the TCNL features themselves. These would indeed by smart materials.