Nuclear-powered rocket engines are not new. In the 1960s, both the U.S. and the Soviet Union developed and tested thermal nuclear rockets fitted with flight-worthy components. However, Project Rover and NERVA (Nuclear Engine for Nuclear Rocket Application) programs were defunded in the early 1970s just before test flights were to start. Now, as part of the Advanced Exploration Systems program at NASA, the Nuclear Cryogenic Propulsion Stage team is tackling a three-year project to demonstrate the viability of and to evaluate materials for thermal nuclear propulsion systems for use in future deep space missions.
A thermal nuclear rocket engine uses a nuclear reactor to heat hydrogen to very high temperatures, with the superheated hydrogen expanding and forced through a nozzle to generate thrust. As such, the performance of a thermal nuclear engine is limited by the high temperature strength of solid materials. Unlike solar or nuclear powered ion or plasma engines, thermal nuclear engines perform largely the same job as chemical rockets (produce multi-g acceleration), but do so with roughly half the fuel requirement.
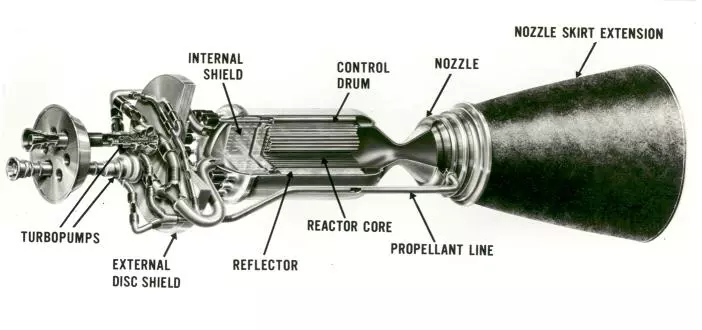
As seen above, a set of turbopumps feed liquid hydrogen into the engine. Most of the hydrogen flow enters the top of the engine enclosure, but part is split off to feed a heat exchanger on the walls of the rocket nozzle, thereby cooling the nozzle and preheating the hydrogen. The turbopumps provide the very large pressure required to keep fuel flowing against the back pressure of the engine.
The hydrogen is fed into the reactor core, which has a matrix of longitudinal channels in which the hydrogen is heated to temperatures in excess of 2500° K. The reactor core is surrounded by a neutron reflector, which allows the use of smaller amounts of fissionable material in the reactor. Once the hydrogen is heated by the reactor, it is exhausted through the nozzle, attaining an exhaust velocity as large as 10 km/s or more.
NERVA (Nuclear Engine for Nuclear Rocket Application)
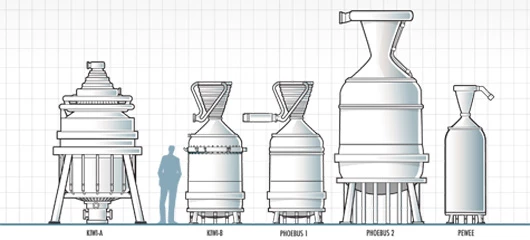
The accomplishments of the NERVA program were considerable. At the very end of the program it had developed nearly flight-ready engines able to operate for periods in excess of an hour while providing maximum thrusts up to 220,000 lb (1000 kN), reactor core temperatures of 2500° K, a specific impulse over 850 seconds (roughly double that of chemical rockets), and a thrust-to-weight ratio of better than 3:1.
An interesting aspect of the NERVA program is how small in physical size they were able to make nuclear engines – reactor cores were about 150 cm (5 ft) in length, and from 50 cm (NRX-A3) to 140 cm (Phoebus-2A) (20 to 55 in) in diameter. Flight tests were upcoming just as the program was cancelled (along with the first U.S. program to put men on Mars).
Nuclear Cryogenic Propulsion Stage
NASA is now taking another look at nuclear engines, primarily for the upper stages of launch vehicles and as primary propulsion for deep space missions. The Nuclear Cryogenic Propulsion Stage (NCPS) is working toward the design and flight validation of a 25,000 lb (100 kN) class thermal nuclear rocket system. As today's materials and production technologies offer considerably better performance than was available 50 years ago, core temperatures and thrust-to-weight ratios are both expected to increase significantly. The NCPS is being designed on the foundations developed during the NERVA program. It will have a fueled weight of about 40 tons, and will be 7.5 m (25 ft) in diameter and 12.3 m (40 ft) in length. It will be launched without the reactor ever having become critical, so that in the event of a launch failure, the only radioactive contaminant will be the uranium fuel (100-150 kg (220-330 lb) of non-weapons grade enriched uranium).
If the NCPS is being used as an upper stage, it will not be started until successfully reaching space. If being used to propel a deep-space craft, the NCPS will not be started until the craft is ready to leave orbit. The development cost is expected to total about four billion US dollars over a 10-12 year period.
NTREES (Nuclear Thermal Rocket Element Environmental Simulator)
The first step toward designing the next generation of thermal nuclear rocket engines is to test and certify new materials with which the maximum reactor core temperature can be increased. To drive this effort forward, NASA has designed and built a facility, called NTREES (Nuclear Thermal Rocket Element Environmental Simulator), that can safely and thoroughly test a wide range of nuclear fuel elements without danger to the environment.
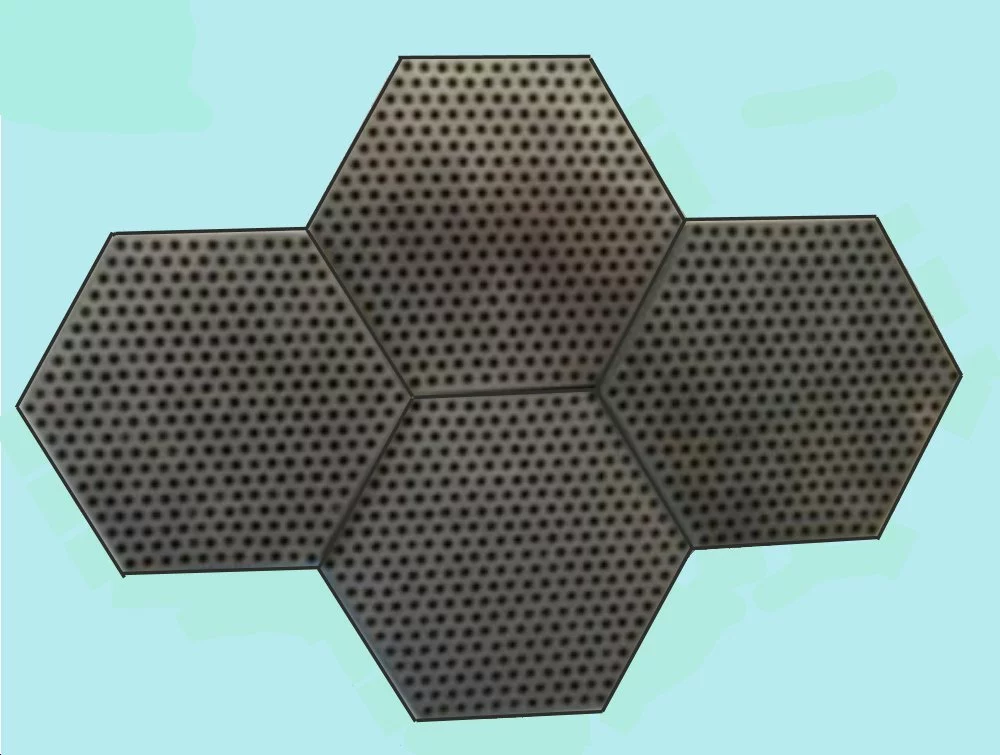
One of the proposed fuel assemblies is made of a cermet (a composite ceramic and metallic material) with a composition of sixty percent uranium dioxide (UO2) and forty percent tungsten metal. It is pierced with about one hundred holes that heat the hydrogen as it passes through the reactor. The holes are about two mm in diameter, and are clad with 90 micron thick tubes of tungsten-rhenium alloy. Each of the hexagonal fuel elements in the assembly is enclosed in a tungsten cladding sleeve 50 microns in thickness.
During the NERVA program it was found that similar fuel elements suffered from reduction of the UO2 into uranium metal that migrated within the cermet, causing structural faults. To stabilize the UO2, five to ten percent of gadolinium oxide was added to the uranium dioxide in the cermet. However, gadolinium is a good neutron absorber, and its use as a stabilizer forces an increase in the size of the reactor. Previous testing used a maximum temperature of 2,750° K, short of the 3000+° K design temperature for the NCPS.
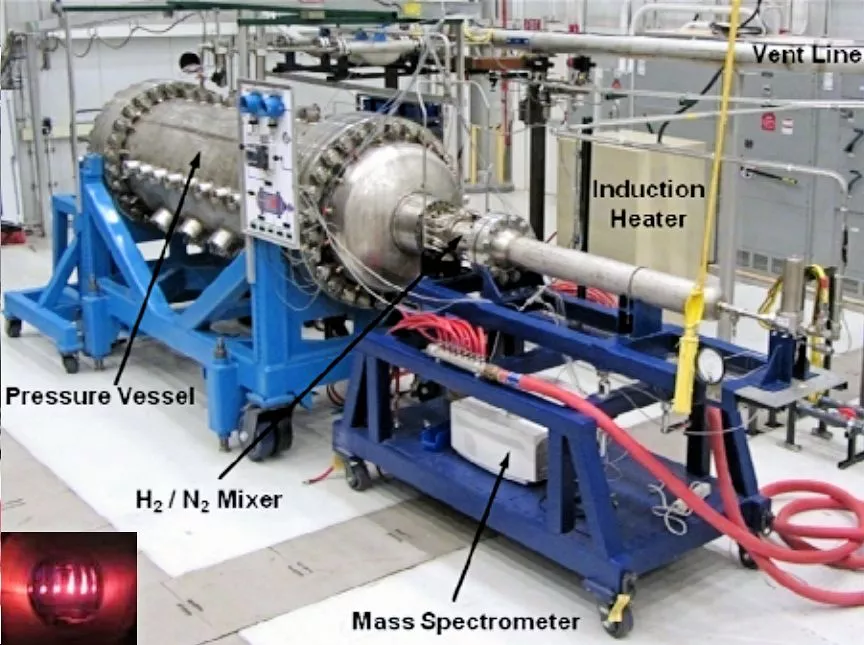
The NTREES facility is designed to test fuel elements and materials in hot flowing hydrogen, reaching pressures up to 1,000 pounds per square inch and temperatures of nearly 5,000° F (2,760° C) – conditions that simulate space-based nuclear propulsion systems to provide baseline data critical to the research team. NTREES is an ideal system in which to study the segregation phenomena described above as well as a host of other potential problems under realistic conditions.
"The information we gain using this test facility will permit engineers to design rugged, efficient fuel elements and nuclear propulsion systems," says NASA researcher Bill Emrich, who manages the NTREES facility at Marshall. "It's our hope that it will enable us to develop a reliable, cost-effective nuclear rocket engine in the not-too-distant future."
The video below shows a short fragment of a NERVA operational test.
Source: NASA, Idaho National Laboratory(PDF)