Ever since Antonie van Leeuwenhoek turned his simple microscope on a bit of pond water in the 17th century, optical microscopes have been a key tool for biologists. Unfortunately, they’re rather limited as to the smallness of what they can see – or at least, they were. This year's winners of the Nobel Laureates in Chemistry, Eric Betzig, Stefan W. Hell and William E. Moerner, changed all that. Their discovery of two methods to bypass the physical limits of optical microscopes led to the creation of the field of nanomicroscopy.
In 1873, Ernst Abbe discovered that there was a seemingly insurmountable limit to how powerful an optical microscope can be. To put it very simply, he found that light can’t get around the bends on tiny objects. According to his calculations, the wavelengths of visible light meant that the maximum limit of the resolution of an optical microscope is 0.2 micrometers, which is about half a wavelength. For over a century, this blocked the lower limit for optical studies, which prevented the direct observation of structures on a nanoscale, such as individual molecules.
What the new Laureates did wasn’t a violation of Abbe’s limit, but more of a workaround. The basic idea was that if light couldn’t be made to bend around nano-sized objects, then the nano-objects could be made to radiate their own light. It’s like the difference between trying to spot a tiny object by shining a giant searchlight on a field, and leaving the field dark but decorating the object with tiny Christmas lights. This insight led to the development of two new methods of what is now called nanomicroscopy.
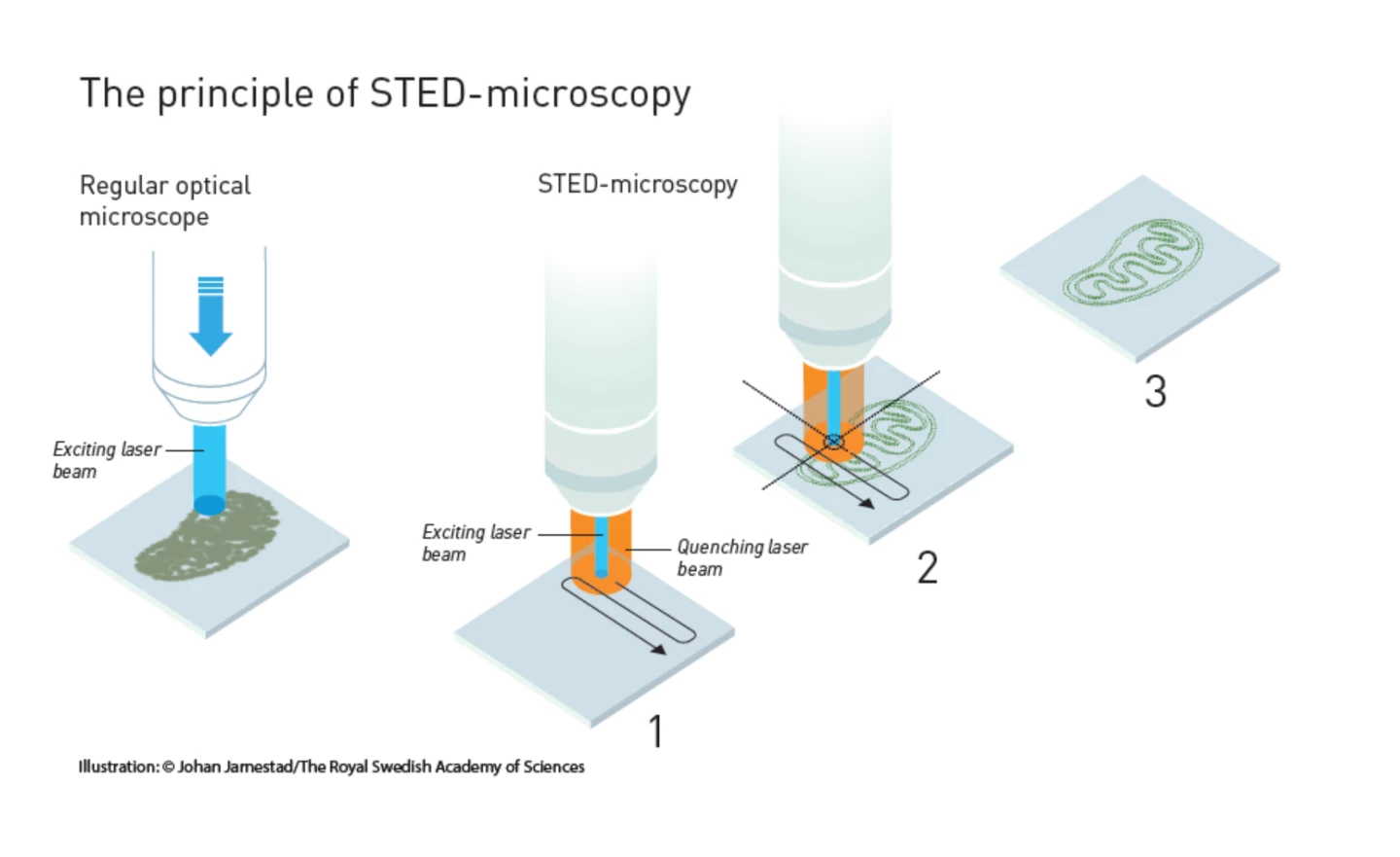
STED Microscopy
The first method is called STimulated Emission Depletion (STED) microscopy, which was discovered by Stefan Hell in 2000, though he’d been working on the problem since his student days in the 1990s. The key to the method was to avoid the diffraction limit by not just using molecules that fluoresce, but to stimulate them to do so using a laser.Hell’s technique used fluorescent molecules, such as antibodies that link to specific structures; for example, DNA strands. When these are hit with a pulse of light, they glow in return. Normally, this produces an image like a cluster of glowing wool, but the STED microscope solves this problem by using a pair of lasers. One sets the molecules glowing, and the other eliminates all but the desired molecules from the picture.
As the first laser scans over a specimen and sets the molecules aglow, a second laser follows close behind. This is tuned so that larger molecules absorb the laser light, causing them to discharge their energy and cease glowing. The nano-sized molecules are too small to be affected and keep giving off light. The result is an image of extremely fine detail.
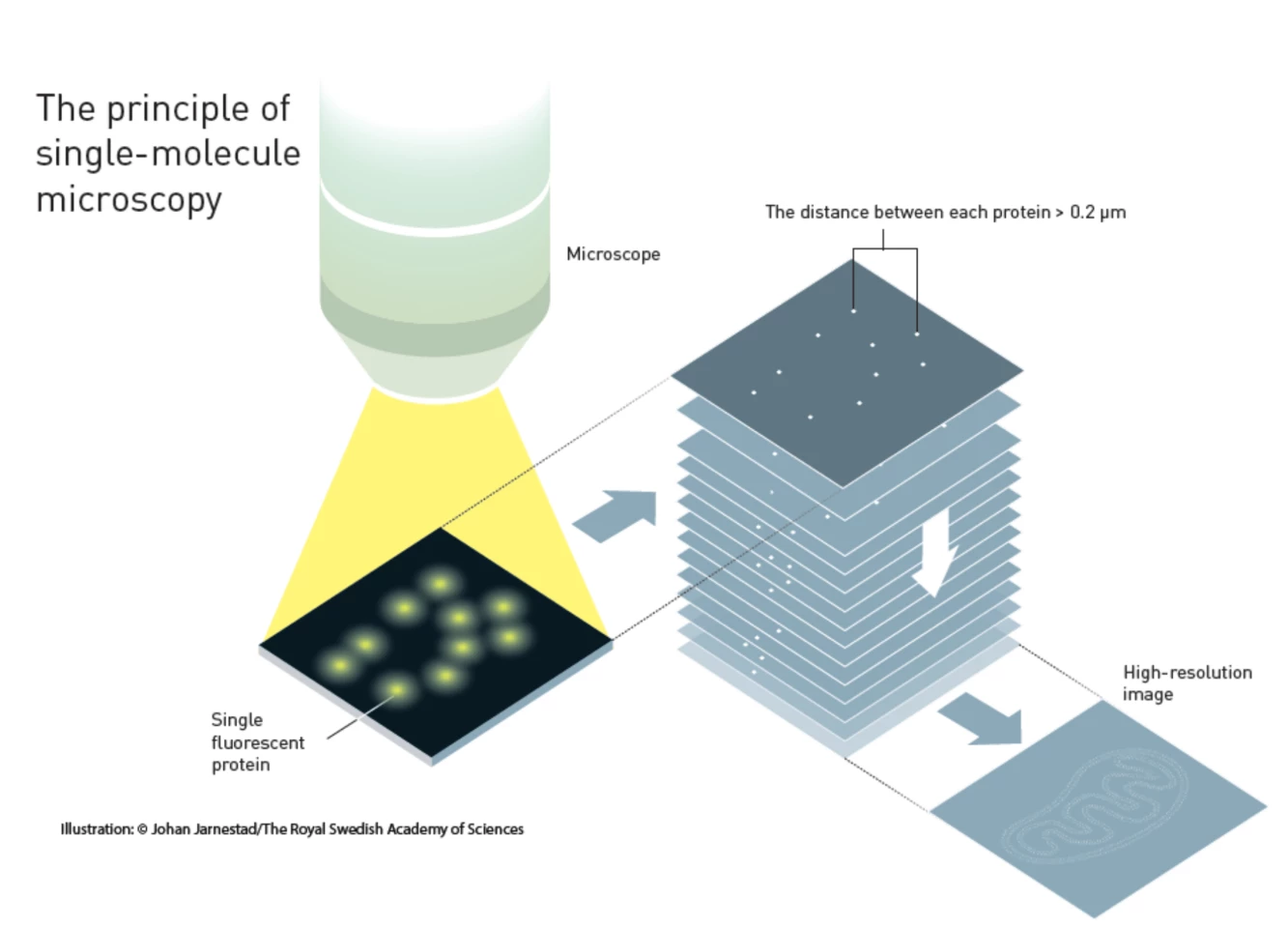
Single Molecule Microscopy
The second method is called Single-Molecule Microscopy. It was developed Eric Betzig and William Moerner while working independent of one another, and was used by Betzig for the first time in 2006. It's based on the ability to see a single fluorescent molecule and using this to build up an image of extremely fine detail.In 1989, W. E. Moerner was the first to measure the light absorption of a single molecule. This was followed in 1997, when Moerner and Roger Tsien at the University of California in San Diego were studying green fluorescent proteins (GFP). Moerner discovered that it was possible to turn this fluorescence of GFPs on and off by using different wavelengths of light. By shining light with a wavelength of 488 nanometers, he could get it to glow, and this would die and not return when shone on again. However, by hitting the molecule with light at 405 nanometers, the molecule would revive and glow again.
This basic idea was expanded upon by Moerner and Betzig independently, to create a new microscopy technique that exploited the on/off system. In this, a specimen would be treated with a number of different fluorescent molecules that bonded to different molecular structures, but always at least 0.2 micrometers apart. Each of these would glow at different times as stimulated. By activating each fluorescent molecule type in turn, then switching them off, a series of different images were produced.
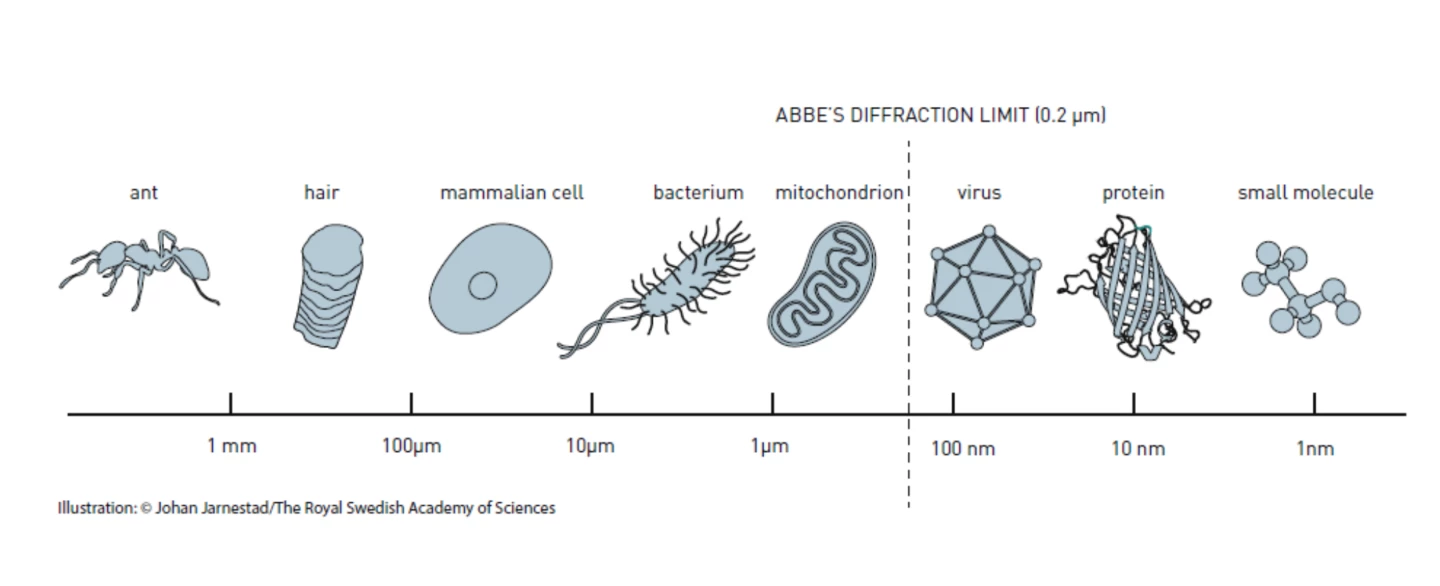
These images were then scanned individually and subjected to a statistical algorithm that sharpened each one. When the images were combined in layers, the result was a single image showing complex, high-resolution structures.
Aside from removing the need for a lot of squinting, these methods have removed the theoretical lower limit to optical microscopy. According to the Nobel Foundation, they are already finding applications, such as the ability to look at individual molecules instead of studying "average" molecules in bulks numbering millions. They are currently being used to study synapses in Alzheimer’s and Huntington’s disease, and to gain a better understanding of protein development in embryos.
Source: The Nobel Foundation