Inside today's computers, information is transferred in the form of bits, a basic unit of measurement that can have a value of either zero or one. In the someday world of quantum computing, information will be transferred via qubits – units as small as photons or electrons held inside some kind of trap. Because qubits can hold a value as both a zero and one, their use could dramatically increase computational speeds. But qubits are notoriously slippery characters and getting them to stay still, or even to exist very long has been a challenge. Now scientists at MIT have figured out a way to get a simple molecule to remain stable for "hundreds of times longer than researchers have previously achieved in these materials," making a usable qubit closer than ever to reality.
To begin with, the MIT team created a simple two-atom molecule out of sodium and potassium. According to Martin Zwierlein, an MIT professor of physics and a principal investigator in MIT's Research Laboratory of Electronics, molecules have a distinct advantage over smaller particles such as atoms or electrons.
"Molecules have more 'handles' than atoms," he said. "They can vibrate, they can rotate, and in fact they can strongly interact with each other, which atoms have a hard time doing. Typically, atoms have to really meet each other, be on top of each other almost, before they see that there's another atom there to interact with, whereas molecules can see each other (over relatively long distances). In order to make these qubits talk to each other and perform calculations, using molecules is a much better idea than using atoms."
Once the molecules were created, the team surrounded thousands of them in a microscopic cloud of gas which was then trapped at the intersection of two laser beams. The next step, though, was the key to the team's success. Molecules were cooled down to around 300 nanokelvins, just a few ten-millionths of a degree above absolute zero.
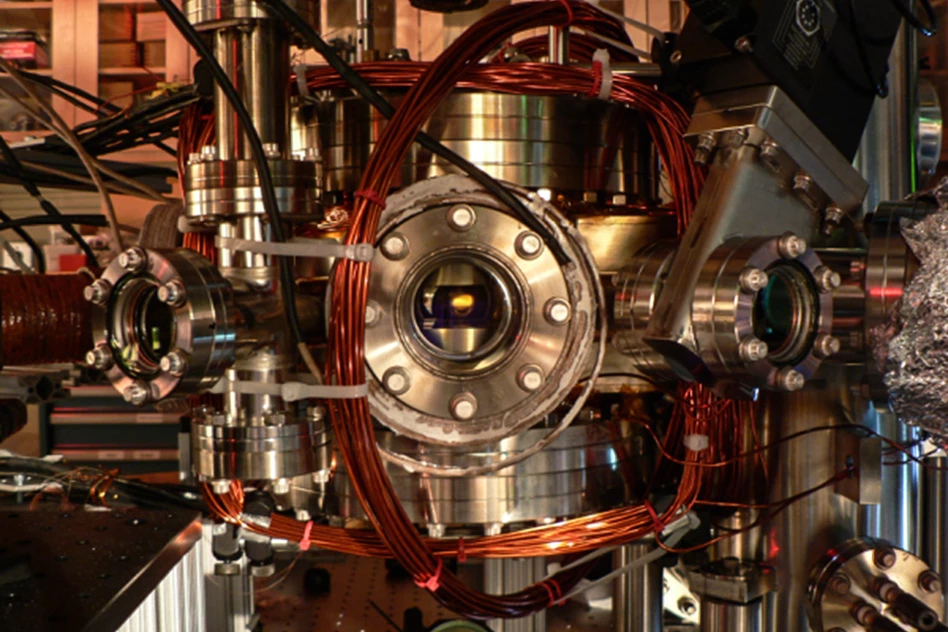
This gave the team control over three important characteristics of the molecules: vibration, rotation and the direction in which the nuclei in each of the atoms could be spun. What's more, the team was able to demonstrate that the molecules at the supercooled temperature could last longer than any previous attempts at keeping qubits coherent. In this case, that equaled only one second, but in the mind-boggling world of quantum computing, that represents a major leap in stability – so much so that a logic gate in a quantum computer that could keep the molecules stable for that long could do an astounding number of calculations.
"We have strong hopes that we can do one so-called gate – that's an operation between two of these qubits – like addition, subtraction, or that sort of equivalent – in a fraction of a millisecond," Zwierlein said. "If you look at the ratio, you could hope to do 10,000 to 100,000 gate operations in the time that we have the coherence in the sample. That has been stated as one of the requirements for a quantum computer, to have that sort of ratio of gate operations to coherence times."
Multiply one sodium/potassium molecule by a thousand and Zwierlein says that a system could perform calculations so complicated that no existing computer could come close.
Of course, such a system is likely a decade or more away. But with work like this, along with advances that have helped quantum computers avoid heating up too much; created a quantum bridge to link multiple computers together; used machine learning to predict and then prevent instability in quantum systems; and created quantum logic gates out of silicon transistors; the "super cool" world of quantum computing is definitely rushing at us faster than you can say "qubit."
"These results are truly state of the art," says Simon Cornish, adding that the results "beautifully reveal the potential of exploiting nuclear spin states in ultracold molecules for applications in quantum information processing, as quantum memories and as a means to probe dipolar interactions and ultracold collisions in polar molecules." Cornish is a professor of physics at Durham University in the UK who was not involved in the MIT research.
"I think the results constitute a major step forward in the field of ultracold molecules and will be of broad interest to the large community of researchers exploring related aspects of quantum science, coherence, quantum information, and quantum simulation," he added.
The work of the MIT team was published today in the journal Science.
Source: MIT