Materials scientists at Harvard University and the University of Exeter have invented a new class of polymer fibers that change color when stretched. As is often seen in nature, the color is not the result of pigments, but rather comes from the interference of light within the multilayered fiber. Inspired by Margaritaria nobilis – also known as the Bastard Hogberry – the new fibers may lead to new forms of sensors, and possibly to smart fabrics whose color changes as the fabric is stretched, squeezed, or heated.
The play of color across a peacock's feathers, the iridescent inner surfaces of certain seashells, the rainbows seen on soap bubbles, or the colors of a fine opal, which seem to float somewhere within the stone – all these are examples of structural colors. In contrast to colors that appear because of absorption of certain wavelengths of light by pigments, structural colors are the result of optical interference of light reflected from nanoscale structures on a surface. Rather like a diffraction grating, the color you see is not that of the substance from which a surface is made, but rather is characteristic of the shape of that surface.
Bastard Hogberry (BH) plays a role in the diet of many birds and of Capuchin monkeys in Central and South America. Unfortunately, its fruit is not very nutritious, so would be shunned by animals who spread its seeds were it not that its bright blue color mimics the fruit of a far superior competitor. The coloring probably evolved as an adaptation – the more its fruit was mistaken for other species, the more widely its seeds were spread.
But where did the color come from? It is harder to make blue pigments through biochemistry than, say, yellow or brown pigments. Blue pigments require an arrangement of high-energy electrons that is expensive for an animal or plant to produce. The excess energy of formation of a blue pigment would have to come from somewhere, which suggests that a jump to a substantially different biochemistry would be required to suddenly start making a blue pigment – an unlikely evolutionary direction.
Nature tends to reuse its abilities whenever possible, and that is the route taken by the Bastard Hogberry. On examining the microscopic structure of a typical BH fruit, the color of the fruit is found to be caused by optical interference of incoming light interacting with a highly complex nanostructure.
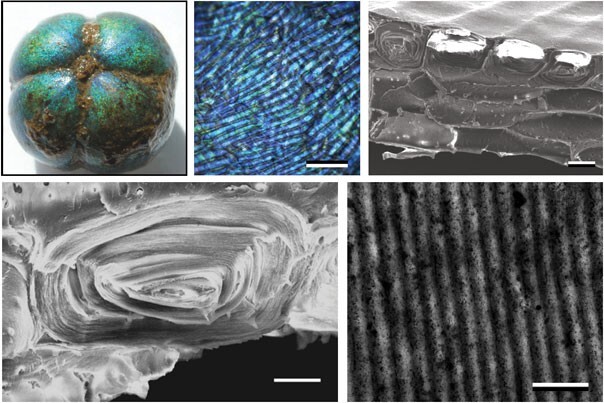
In the figure above is a BH fruit as it appears at a wide range of magnification. The upper left photo is of a single BH fruit about one cm in diameter. The upper center photo shows a section about a millimeter across, and shows strips of blue cells. On the upper right is a scanning electron micrograph about 100 microns across, showing a cross-section of the outer layers of the fruit revealing layers of cells.
At the bottom left is a view about 50 microns across showing a cross-section of a single tissue cell, revealing its concentric, slightly flattened cylindrical multilayer structure. Finally, at bottom right is a 2-micron wide view showing details of the stacked layers. In this final image it becomes clear that the layers are actually bilayers – one material upon another, and that bilayer then makes up the cylindrical multilayer structure of the hard inner layer of the BH fruit.
This was the key observation. The fruit cells look like a periodic stack of bilayers from every direction. As each of the materials making up the bilayers has a different index of refraction, the multilayers form Fabry-Perot dichroic filters which reflect one characteristic color. The color of the light depends on the thickness of the bilayers and the difference of optical properties in the two materials making up the bilayers.
In the case of BH fruit, the fibers reflect blue light and transmit other colors to be absorbed in the brown underlying plant material. The color is the same from any angle around the axis of the cylinder, but varies when the viewpoint is not perpendicular to the cylinder axis. This leads to the iridescence of the blue color.
“Our new fiber is based on a structure we found in nature, and through clever engineering we’ve taken its capabilities a step further,” says lead author Mathias Kolle, a postdoctoral fellow at the Harvard School of Engineering and Applied Sciences (SEAS). “The plant, of course, cannot change color. By combining its structure with an elastic material, however, we’ve created an artificial version that passes through a full rainbow of colors as it’s stretched.”
To make the artificial color-changing photonic fibers, the members of the Harvard-Exeter team mimicked the optical structure of the fruit cells using an novel roll-up mechanism perfected in the Harvard laboratories.
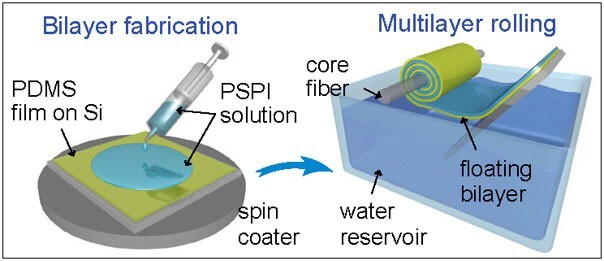
“For our artificial structure, we cut down the complexity of the fruit to just its key elements,” explains Kolle. “We use very thin fibers and wrap a polymer bilayer around them. That gives us the refractive index contrast, the right number of layers, and the curved, cylindrical cross-section that we need to produce these vivid colors.”
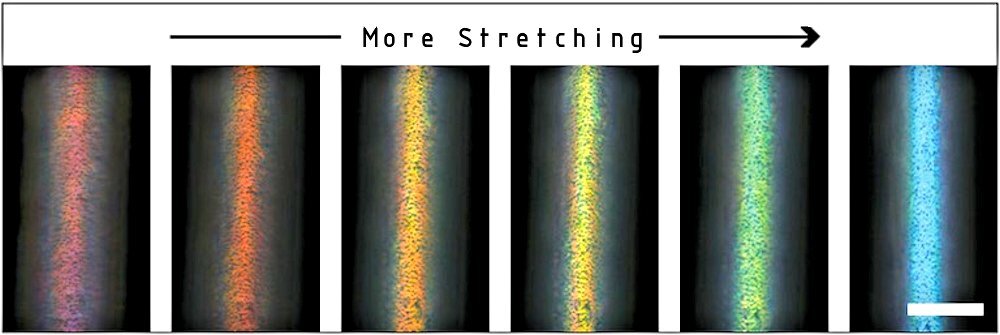
As you can see above, the result was quite successful. But why does the color of the fiber change as it is stretched? Think about stretching a rubber band. As you do, the rubber becomes thinner and narrower. The more you stretch, the thinner it becomes. This phenomenon is called the Poisson effect, and to some degree occurs in most elastic materials.
As one of the new fibers is stretched, the bilayers become thinner, so to keep the optical conditions that produce the fiber color the same, the reflected color will shift to having a shorter wavelength. If an unstretched fiber has a red color, stretching the fiber will change its color to orange, yellow, green, and then finally blue as seen above. In that example, the wavelength becomes about 35 percent shorter as the fiber's length is doubled by stretching.
Other optical effects occur in the new fibers, based on the same principle. For example, if you apply pressure perpendicular to the fibers to compress them, two things happen. One is that the bilayers that are being squeezed become thinner, so their color in the direction of the pressure moves toward the blue. On the sides, however, the bilayers actually become a little thicker, which moves the color perpendicular to the pressure toward the red.
So, pressure causes the fiber colors to shift with the viewing angle, an effect that could be fascinating in a "smart cloth" made of these photonic fibers. The color will also change with the temperature of the fiber, due to thermal expansion. Higher temperatures cause a shift to redder colors.
“Our fiber-rolling technique allows the use of a wide range of materials, especially elastic ones, with the color-tuning range exceeding by an order of magnitude anything that has been reported for thermally drawn fibers,” says coauthor Joanna Aizenberg, Amy Smith Berylson Professor of Materials Science at Harvard SEAS.
Athletic spandex that change color as you move? Superhero costumes whose color changes to tell you who is winning the latest battle? Adaptive camouflage that can change patterns by controlling a heating grid within the fabric? Or (more likely), packaging materials whose color tells you if the wrappings are about to burst? There are a wide range of applications for this new class of photonic fibers just awaiting further development and commercialization. I can't wait!
Source: Harvard University