An international team of researchers at the US Department of Energy's Jefferson Accelerator Laboratory in Virginia has measured the weak charge of the proton for the first time. Although first measurements of fundamental quantities always raise the hope of observing new physics, early results from the Q-Weak experiment find the weak charge of the proton and the neutron to be consistent with predictions of the Standard Model. Analysis of additional data will help pin down this result.
The well-named weak interaction between particles is so weak that neutrinos (particles that interact only through the weak interaction and gravity) pass easily through a light-year of lead without interacting with any of the lead nuclei.
Particles have a weak charge, analogous to the electric charge, that describes a particle's propensity to have weak interactions with other particles. This weak charge is predicted by the Standard Model to be about -0.989 for neutrons and about + 0.071 for protons (+) and electrons (-), as measured in elementary charges.
The weak interaction allows particle-particle interactions that violate parity, a symmetry closely related to mirror symmetry. As none of the other fundamental interactions violate this symmetry, an experimental handle is thereby provided with which to separate out weak interaction effects which would otherwise be swamped by stronger effects from the other interactions.
This can be seen clearly in Chien-Shiung Wu's beta-decay experiment, carried out in 1956, that first directly demonstrated violation of parity symmetry in weak interactions. Beta decay of a radioactive nucleus occurs when a neutron changes into a proton. This type of decay occurs through weak interactions.
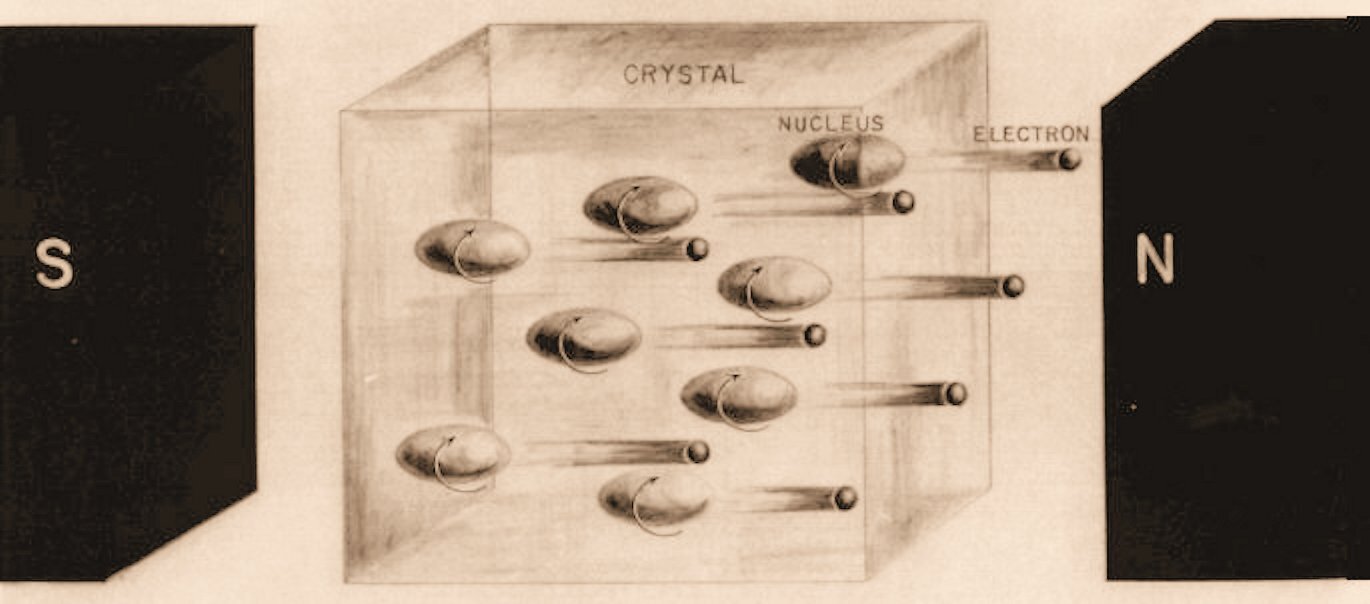
Wu's experiment looked at the angular distribution of electrons produced by beta-decay of cobalt-60. The beta rays (electrons) are generated by the weak interaction, while the gamma rays are generated by the electromagnetic interaction. It had already been demonstrated that electromagnetic interactions conserve parity, so if there appeared any difference in the angular distribution of the gamma rays and of the beta rays, it would be a clear sign that parity had been violated. When Professor Wu carried out the experiment, she observed a huge difference between the distribution of the beta and gamma rays, demonstrating that the weak interaction violates parity in a big way.
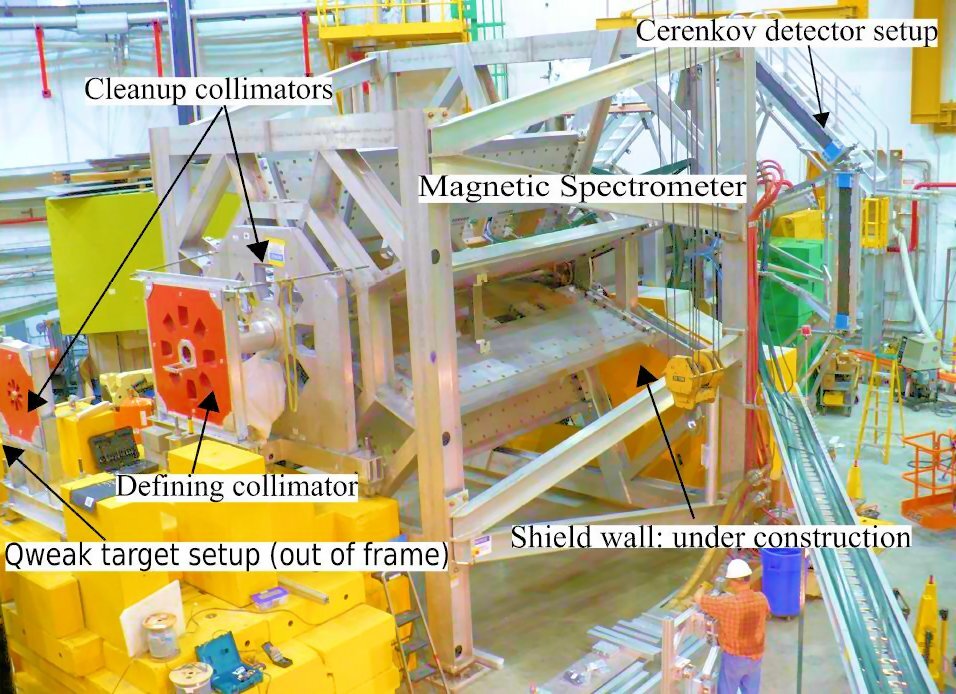
The recent Q-Weak experiment determines the weak charge of the proton by analyzing the scattering of a beam of polarized electrons from the nuclei of a liquid hydrogen target. The tricky bit is that electrons and protons (the hydrogen nuclei) interact through the electromagnetic interaction far more strongly than through the weak interaction, at least until particles are only separated by an attometer (10
m) or so. As a result, most of the scattering observed is due to electromagnetic forces.Only that part of the scattering caused by the weak interactions will violate parity. In this case, that is about one in five million – clearly not something you can observe with the unaided eye. The Q-Weak experiment seeks to isolate this tiny portion for analysis by reversing the polarization of the electron beam. Reversing the polarization produces exactly the same average electromagnetic scattering, but the weak interaction scattering is reversed in momentum. Given large enough numbers of electrons scattering off hydrogen protons, the weak signal can be isolated statistically, and its magnitude measured with a good deal of accuracy.
The results just announced are preliminary, only taking into account four percent of the total amount of data obtained in the experiment. However, that is sufficient to establish an experimental value for the weak charge of the proton of 0.064 + 0.012 elementary charges. This is sufficiently accurate to also set the neutron's weak charge at -0.975 + 0.010. Both numbers are quite consistent with the Standard Model predictions of 0.071 and -0.989. When the entire data base is examined, these charges should be set experimentally to better than five percent, a remarkable accuracy for the first measurement of such a difficult constant.
What about new physics? At the moment, there is still room for a significant deviation between the predicted and measured weak charge of the proton, but that result would require rather special behavior of the remaining data, so it seems unlikely. The most likely result appears to be that the Standard Model does a very good job in predicting weak charges of elementary and composite particles.
The surprising part of this conclusion, if it survives the onslaught of more data, is that we know that the treatment of weak interactions in the Standard Model is, if not simply wrong, rather incomplete. Neutrinos are predicted to have no mass, whereas it is well known from observation of neutrino oscillations that they must have a mass (albeit a tiny one). There are modifications of the Standard Model to add this property in by brute force, but these are in the long run unsatisfactory. At present, though, it appears that the weak charges of particles is not where the Standard Model breaks down. Hopefully that elusive Achilles' heel will soon be discovered.
Source: Jefferson Accelerator Laboratory