Among the mechanical components not found in nature is the spur gear. That is, until now. Zoologists of the University of Cambridge have discovered that the juvenile form of the leaf-hopper Issus coleoptratus has a set of gear-like linkages between the two jumping legs to synchronize the legs during a jump.
Engineers and scientists have often turned to biomimetics, the imitation of nature's solutions, in their attempts to manipulate and control the Universe. Armor is inspired by scales and shells, Velcro by the burrs of plant seeds, and surgical glue by barnacles. In reverse, however, there are mechanisms invented by humans that received little if any sampling during the evolutionary process. For example, the only close biological analogs of the wheel and axle are the flagella of individual cells, which are driven by a rotary molecular motor.
The evolutionary fitness of a leaf-hopper to some extent depends on how successful it is at accessing a food source while expending as little energy as possible in the attempt. In accord with this principle, leaf-hoppers do not simply jump toward a bush, but rather select a target that provides the best sensory evidence of being a tasty, yet safe, dinner.
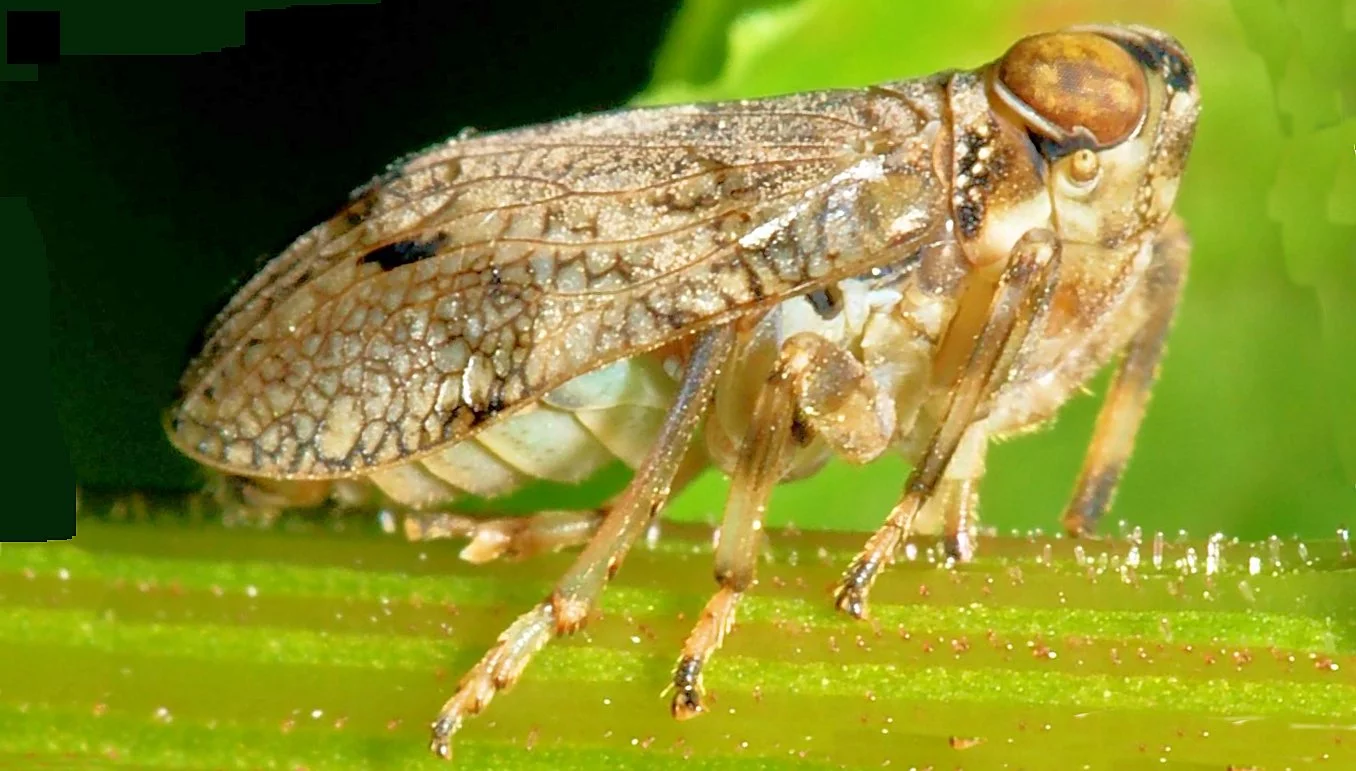
Issus leaf-hoppers are generally about a quarter-inch (6 mm) in length, and can make jumps of nearly a meter in height. To do so, their rear legs generate a remarkable 200 g acceleration for a period of about two milliseconds, requiring generation of a propulsive force of about an ounce.
An insect that jumps for a living is better off if those jumps take it exactly to a food source it has spotted. That is not going to happen reliably if the motion of the two jumping legs is not closely synchronized. If not synchronized, the unbalanced force from the first leg to push would induce a torque around the center of gravity of the insect, driving the insect off-course and spinning in an uncontrollable manner.
The time scale of the nerve impulses that trigger the independent jumping muscles of the legs is about a millisecond, so effective synchronization cannot depend simply on timing of the nervous system – it simply doesn't act quickly enough. In most jumping insects, synchronization is aided by frictional contact between the rear legs. Indeed, this is how the adult Issus insures synchronization at even larger accelerations.
In the juvenile Issus, however, nature has utilized a different approach to the problem of synchronizing the efforts of the insect's legs.
Professor Malcolm Burroughs of the University of Cambridge zoology department and his student Gregory Sutton (who is currently a post-doc at the University of Bristol) have discovered that tiny strips of gear teeth appear on the opposing inner surfaces of the jumping legs, which mesh together during a jump, thereby providing mechanical synchronization for an accurate jump.
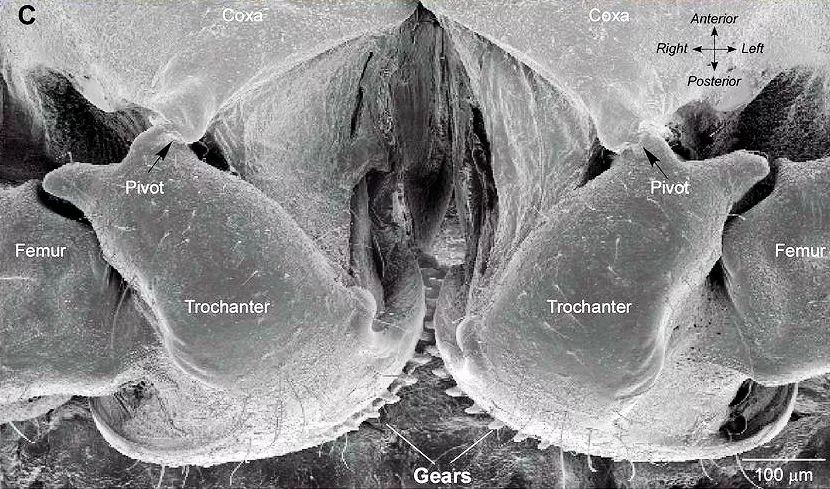
These gear strips are about 0.4 mm (15 mils) in length, forming a circular segment of a gear wheel about 0.4 mm in diameter. Each strip comprises 10 to 12 gear teeth, each averaging about 50 microns (2 mils) in width and 15-30 microns (0.6-1.2 mils) in length, with a thickness of about 9 microns (0.4 mils). A complete circular spur gear of the same dimensions would have about 32 to 38 teeth.
The teeth are formed of the chitinous material of the insect's exoskeleton, modified so that the chitin forms a tough composite material with sclerotin, a cross-linked protein-based polymer whose mixture results in a hard, horny material. The shape of the teeth is asymmetric, so that they only link the motion of the legs together when driving a jump. At other times the legs can move largely independently of each other.
The gear teeth are highly asymmetric in shape, a feature that improves the endurance of the teeth as long as high stresses are only encountered in one direction of rotation, for example, during the take-off jump of Issus. Asymmetric gear teeth are also used by engineers, but rather sparingly as they require special tooling for manufacture.
The dimensions of the Issus gears show that synchronization of the jumping actions of the insect's legs can be fixed to within a few tens of microseconds – perhaps an order of magnitude better synchronization than would result from using nerve impulses alone for that purpose. This is a remarkable adaptation, but unlike many such, it is relatively easy to put together a scenario in which such gears might have evolved.
It seems likely that an ancestral form of Issus would have used friction between the legs to aid in synchronization, as do other jumping insects. Issus, however, performs jumps far longer relative to its size than do most insects, so the smaller area available to generate friction may not have been sufficient for effective synchronization.
One way to increase friction between two surfaces is to roughen the surfaces, thereby introducing barriers to slippage. Such mechanical obstruction is not conventional friction, but acts as if it were, providing stronger linkages between the two surfaces. There will always be variations in surface smoothness within a species, and if such variations improve the chance of survival, they will persist in the genome of the species and propagate to future generations.
Once surface roughness proves to be a survival characteristic, the natural variation within the species will change from "smooth to fairly rough surfaces" to "somewhat rough to very rough surfaces." As long as still rougher surfaces lead to improved food gathering, they are likely to be favored by the evolutionary process. However, a very rough pair of surfaces can actually have smaller sliding friction if the surface features are not correlated in some manner. Without such correlation, the benefits of mechanical obstruction tend to vanish.
As a result, the next direction of variation to consider is patterning the surface roughness. If patterns of roughness that improve the interlocking of the two surfaces result in better jumps (and hence better survival), such patterns will be favored in the evolutionary process. Gear teeth are just a highly refined form of patterned roughness that can evolve from smooth surfaces without any uncertain gaps to leap.
Having said that, it is also clear that most insects do not gain any benefit from gear teeth, or they would be common instead of vanishingly rare. The difference is probably the gigantic leaps taken by Issus. Insects which are larger, or take less prodigious leaps, may do just fine with friction helping their jump synchronization, and in the absence of an improvement in survival, features are unlikely to evolve very far from their simplest form. In the case of the juvenile Issus, however, the evolutionary advantage seems to be significant.
Interestingly, the adult Issus loses its gear teeth, returning to the slightly rough surfaces which are common among insects. The authors of the study suggest that the distinction may be in the amount of time over which the gear teeth must function. The juvenile forms molt regularly, forming new exoskeletons as they grow in size. As a result, the gear teeth don't have to last very long.
In contrast, the adult form must rely on its jumping mechanism for a much longer period of time, as it is stuck in its final exoskeleton for the rest of its life. Further, as the adult form (by definition) is no longer growing, it should require fewer calories to remain healthy, a pattern seen in most animal species. Accordingly, the survival advantage of precision jumps may be overcome by serious problems that might arise from damaged or broken gear teeth which cannot be replaced.
A great deal of additional study would be required to firmly embed this "just-so" story in evidence. However, even a plausibility argument helps understand that these unusual features could have evolved, even if the exact path through which they did evolve remains unclear.
The discovery of gear teeth in the animal kingdom, while remarkable, fits nicely into the pattern of evolution driven by differential survival of a species. It underscores the amazing flexibility of nature in exploiting every possible niche of structure and mechanism for the purpose of survival. In this case, understanding does not reduce the sense of wonder at the natural universe.
Source: University of Cambridge