While not a primary source of energy, hydrogen, because of its large energy density, provides a vehicle with which to store and transport energy. Photoelectrochemical (PEC) cells can use sunlight to sustainably split water into hydrogen and oxygen, but efficient PEC materials tend to corrode rapidly in use. A Stanford research group has been studying this problem, and has found that depositing a thin layer of nickel atoms on a silicon PEC electrode allows it to operate for over 80 hours with no sign of corrosion.
If you want to harvest the energy from sunlight on a large scale, looking into using silicon would be a good start. Cheap and workable owing largely to the massive infrastructure built to support the fabrication of integrated circuits, silicon can absorb from the near-IR into the UV, a range that covers the peak wavelengths of the Sun's radiation. Silicon solar cells have attained as much as 30 percent conversion of sunlight into electricity.
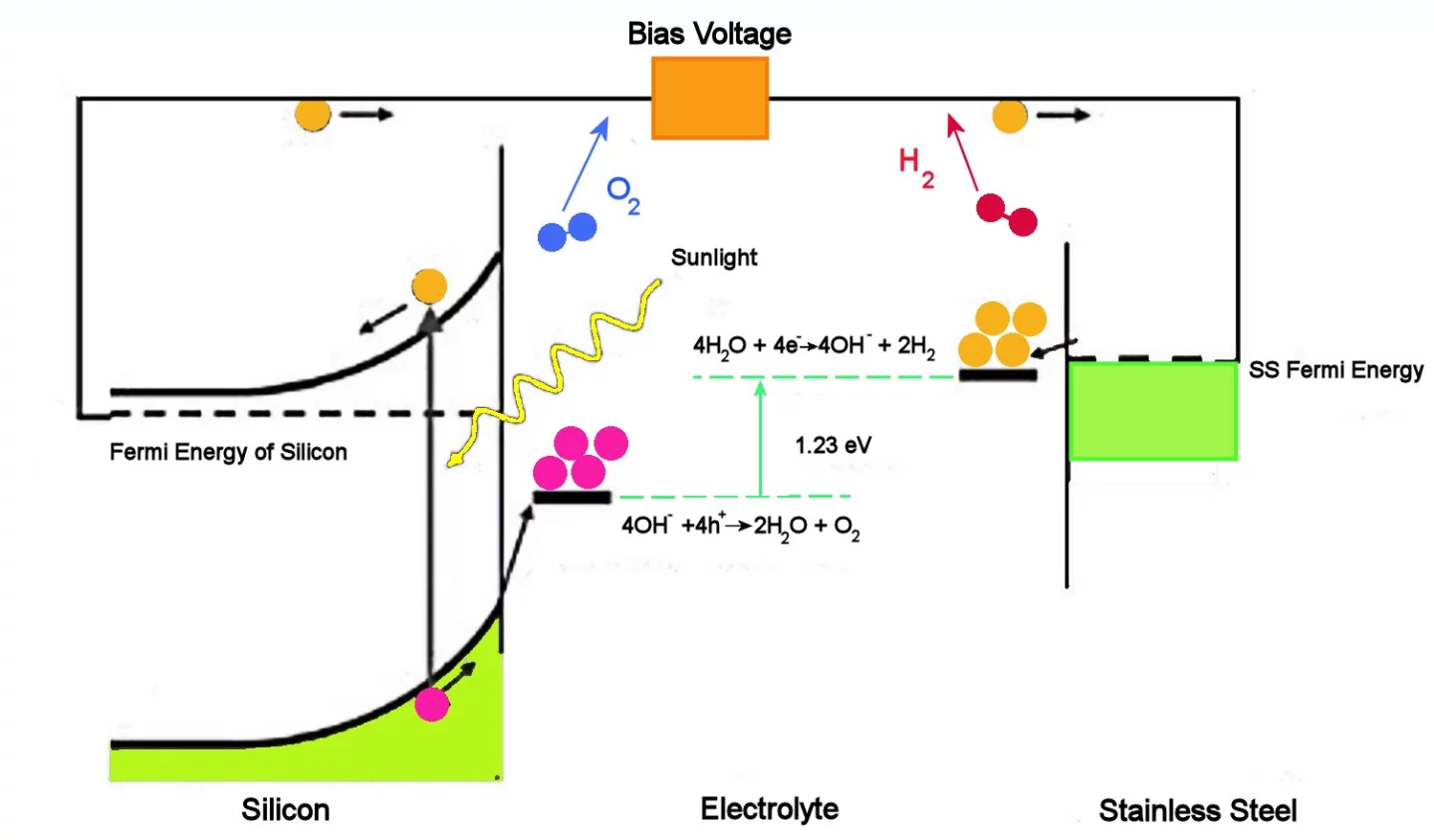
The figure above illustrates how a PEC cell operates. Briefly, incoming sunlight excites free electrons near the surface of the silicon electrode. These electrons are free to flow through the wires to the stainless steel electrode at the other side of the cell, where four of them react with four water molecules to form two molecules of hydrogen and 4 OH groups.
The OH groups flow through the liquid electrolyte to the surface of the silicon electrode. There they react with the four holes associated with the four photoelectrons, the result being two water molecules and two oxygen molecules. Notice that while electrons do not have to travel through the electrolyte, the OH groups do.
Only things aren't quite that simple in practice. In particular, illuminated silicon begins to corrode immediately when in contact with the electrolytes used in PEC cells. Not only does corrosion eat materials, but the operation of a PEC cell to generate hydrogen depends sensitively on the properties of the surfaces and interfaces within the cell. If chemical reactions are changing the materials of which those features are formed, the odds are tiny that the result will be an efficient PEC cell.
The lifetime of practical PEC cells must be long enough to recover construction costs and minimize routine maintenance. This requires a lifecycle measured in thousands (if not tens of thousands) of operating hours. Prior to the work being described here, eight hours of operation without noticeable corrosion was considered a significant advance, indicating a good deal of room for improvement.
This eight hour silicon electrode was protected from the electrolyte by a surface layer of titanium dioxide and iridium. Stanford professor of chemistry Hongjie Dai thought that a thin (2 nm) nanolayer of nickel, also a metal well known as resistant to corrosion, might perform better.
So his group tried it out. They built a PEC cell with 2 nanometers of nickel on a silicon electrode, paired it with a stainless steel electrode, and immersed both in a solution of potassium borate and lithium borate in water (introducing lithium in electrolytes also helped Thomas Edison in his quest for better batteries). Fresh electrolyte was introduced into the cell twice a day to avoid changes in concentration.
The resulting PEC cell split water for 80 continuous hours with on sign of surface corrosion. In the process, about 150 ml of hydrogen gas was generated, representing the storage of about 2 kilojoules of energy.
The result points out a new path toward longer-lasting PEC cells. "Our lab has produced one of the longest lasting silicon-based photoanodes," Dai said. "The results suggest that an ultrathin nickel coating not only suppresses corrosion but also serves as an electrocatalyst to expedite the otherwise sluggish water-splitting reaction." In an area of study in which "breakthroughs" seem to appear monthly, hopefully this new path will be a path toward a solution, rather than a dead end.
Source: Stanford University