Nano-engineering happens all day long in our bodies, and California startup Aether is designing and testing millions of new enzymes to do a range of other useful tasks – like directly extracting battery-grade lithium from sources nobody else can use.
These enzymes can be designed to selectively grab more or less any particular atom or molecule, and either split it into constituent parts, force it to bond to a different molecule, or simply catch and release it on command.
What you end up with is a bunch of totally tunable molecular factories that can take a given input and transform it into a particular output, replacing all manner of chemical manufacturing processes with new versions that can be incredibly cheap and energy-efficient, because they don't require heat.
And beyond that, there's also the opportunity to create entirely new compounds that chemistry hasn't been able to synthesize. New chemicals, materials and molecules with unprecedented properties.
Because many of these enzymes are entirely new and don't exist in nature, Aether is creating them in enormous numbers, and rigorously testing them to determine their effects on a range of other elements and molecules, generating great scads of data and feeding it all into custom-trained machine learning algorithms to catalog the effects.
And it's already got runs on the board. Aether co-founder Pavle Jeremic told us over a video call that the company has designed a class of enzymes that can capture lithium atoms straight out of low-concentration brines that nobody else can economically harvest, and release them to create pure, battery-grade lithium – at a cost far below what traditional methods can deliver.
Over the next couple of years, the company plans to prove this tech and develop a molecular lithium mining business – and use the profits to expand out into all sorts of other areas, with the eventual goal being to build a "post-scarcity future" of rampant abundance, in which all kinds of raw materials are available at radically lower costs, and in which complex and novel molecules can be synthesized quickly and cheaply.
We spoke to Jeremic over a video link. What follows is an edited transcript.
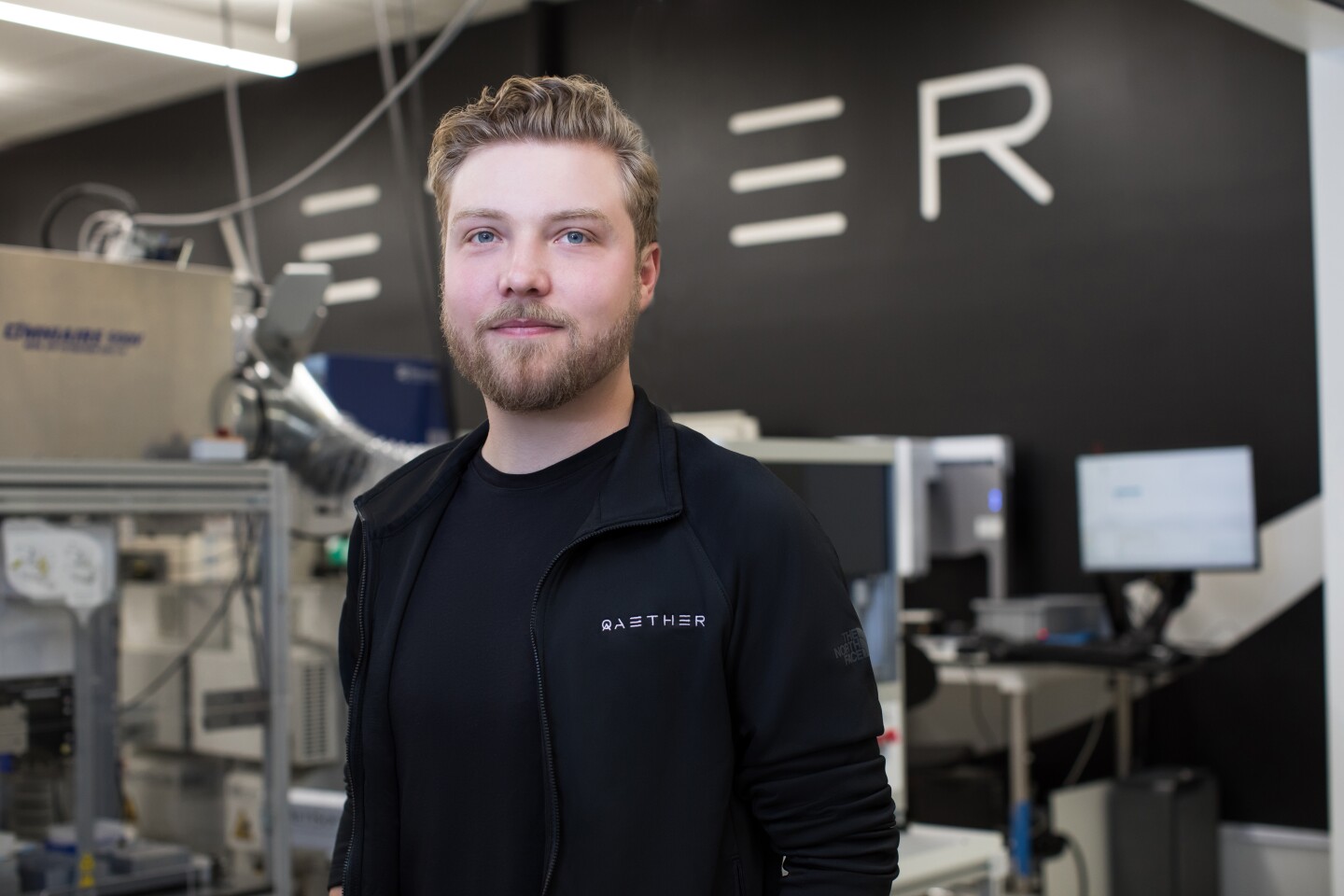
Loz: I heard an interview somewhere in which Elon Musk talks about the nature of manufacturing from a first principles perspective. As in, you've got a pile of atoms in one formation and you want them in another, in the simplest, cheapest and most efficient way.
Pavle Jeremic: Yeah, I mean, the goal of what we're trying to build is technology to assemble molecules. Really to assemble any pattern of atoms you want, eventually.
The way I like to think about it is, we've seen what the human race is able to do by patterning silicon in just two dimensions. So what if we can pattern more than silicon – and more than two dimensions, right? What can we start to build? What kind of technology could become possible that simply isn't possible today?
And you're using enzymes, some of which exist in the body naturally – in a basic sense, how do they work?
You can think of an enzyme as a very, very small machine. It's designed to recognize and grab on and hold on to certain molecules or arrangements of atoms, and then either break them apart or make them form some new pattern.
A useful metaphor is when you look at how our species has traditionally manufactured things. It's a very sort of brute force, stochastic process. And so for example, when you're looking at chemical manufacturing, two atoms are only going to want to form a bond when they come together at a certain angle and a certain velocity, effectively. Otherwise, they won't want to form bonds.
And so as a species, what we've done very successfully is we've figured out, like, in some situations, if we heat up a reaction enough, the atoms will hit each other often enough at that specific angle, they'll be able to make the molecule we want. It's kind of like assembling a Lego kit by shaking the box until it's properly assembled. You know, statistically, if you shake the box enough, it will eventually happen. And that's kind of what we do as a species, and we've made that work.
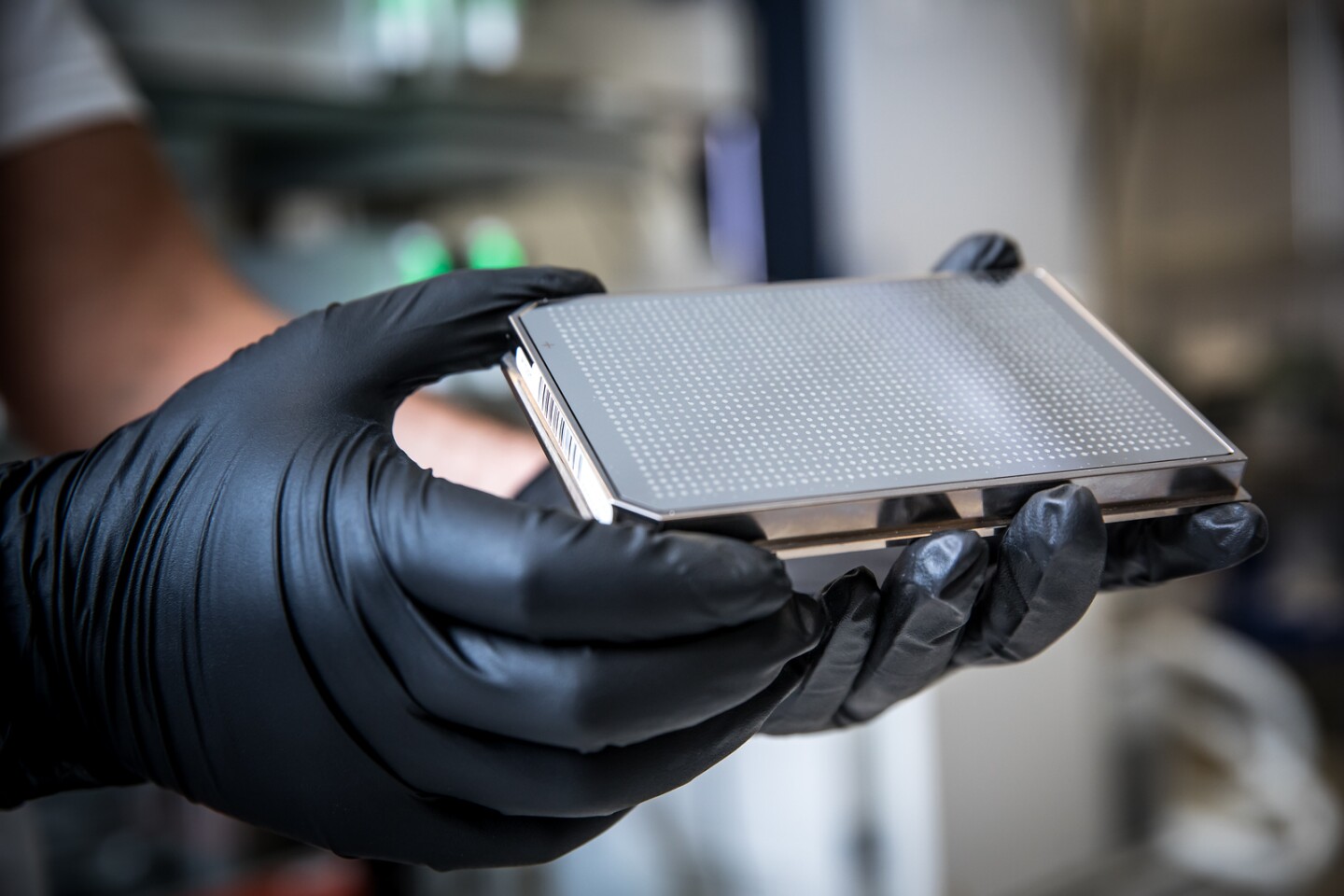
Enzymes, and what biology does, is very different. At that scale, rather than relying on these sort of random interactions – well, there's still randomness to it, of course – but it's very much machinery at the nanoscale. If you want atom A and atom B to form a bond, the machine floats around until it sees an atom A, grabs onto it, floats around until it sees atom B, grabs onto it, and then forces them to form a bond. So it's a very mechanical process, as opposed to this sort of random, stochastic process that we use at industrial scale.
And so what we're doing at Aether is very much inspired by these enzymes, but going beyond what nature has invented, in a lot of ways. You know, how do we start with some things that nature invented, but go beyond that, and build truly functional molecular assemblers where you could just eventually specify an input arrangement of atoms and an output range of atoms. And we could figure out what is the machine that we can engineer to make that rearrangement balance happen with high efficiency.
And the benefits of that? Certainly there's environmental benefits. Even like what I mentioned before, you don't have to heat up your reaction to make the reaction go through, it requires less energy, requires fewer toxic pollutants, all that kind of stuff. That's one side of the coin, which is true.
But the other thing which I think is actually even more valuable is when you look at the kinds of molecules, and the kinds of arrangement of atoms that become possible if you really have this kind of generalizable technology. I think it's not just about making things more abundant. It's about creating products that have properties that are simply unprecedented today.
For example, we have a product line where we're creating basically a new type of polymer that's designed so that before you manufacture the polymer – before you melt it into a mold, or 3D print it, or you know, whatever your preferred manufacturing process is – it's designed to be highly malleable, which makes it very easy to mold into very complex shapes.
But the moment you heat it, it changes its microstructure. So it's actually programmed to completely rearrange itself and it becomes incredibly tough. And it creates like a nanoscale composite basically. And this molecule is basically impossible to make using traditional synthetic chemistry. But a lot of the machinery that we've designed allows us to make these molecules and then create products based off those molecules.
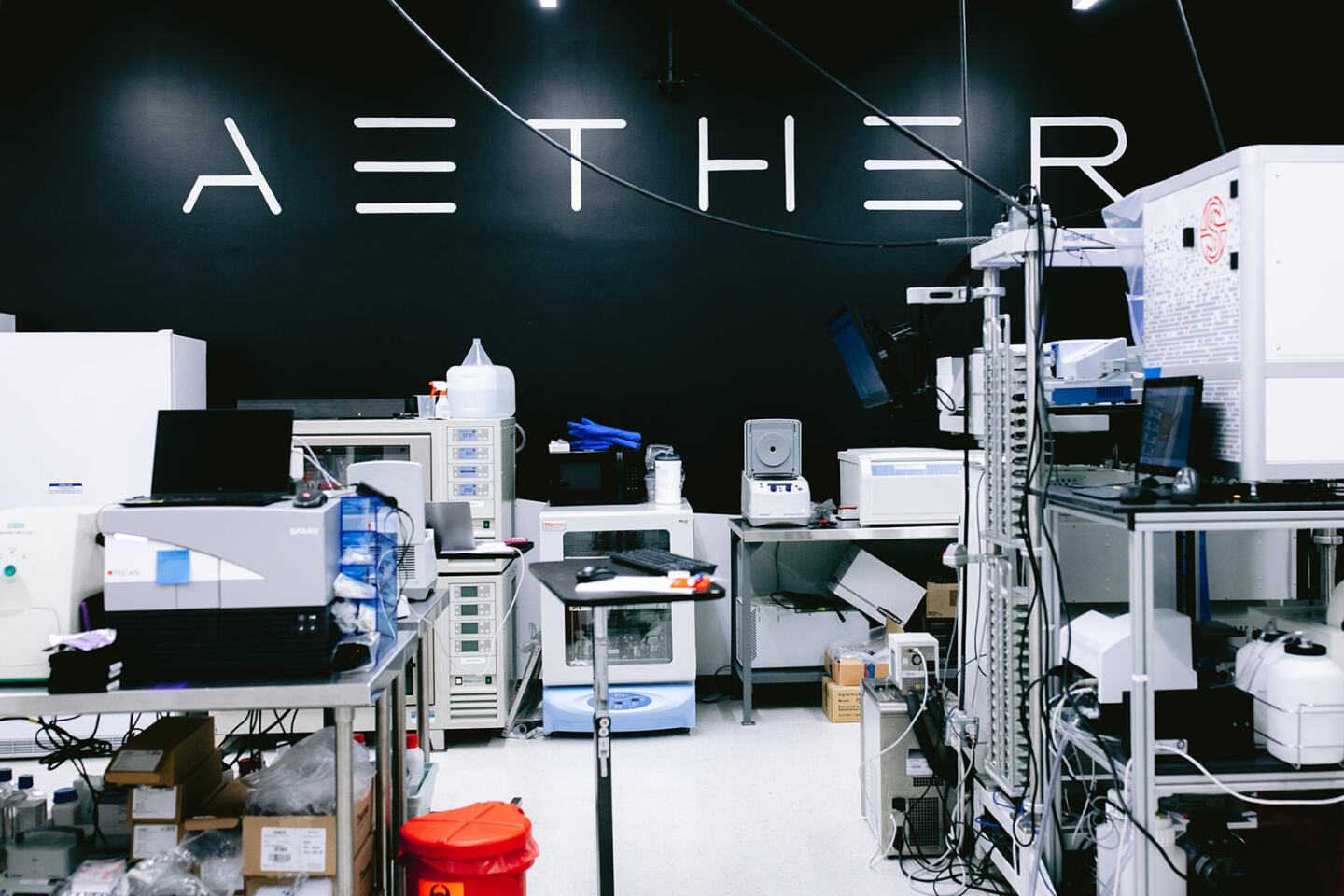
Remarkable. So the basic idea for you guys, as I understand it, is "let's throw a whole bunch of shit at the wall, essentially, let's do millions and millions of tests of different enzymes with other components. See what happens. Put it in some giant database somewhere, and analyze everything using machine learning to try and figure out which ones do what to whom?
That's exactly right. The machine learning is trained off data where you test a huge number of these enzymes and other proteins. Honestly, we have to come up with a better term for these, because they don't really look like anything in nature anymore. They're mostly made of amino acids like proteins, but there's things about them that are just totally different than what exists in nature.
But these molecular machines, we test a huge number of them and what the machine learning is trying to figure out is okay, I know what arrangement of atoms I started with. I know the machine that I'm testing. So what's the new arrangement of atoms that came out of the back end? And it starts to see patterns; okay, this kind of machine allows me to create this type of polymer. This type of machine allows me to degrade this toxin that we can't currently degrade. This one will bind to metals, right?
A lot of our mineral work is based off of these tiny, tiny little nano-proteins. We've designed them so that with lithium, for example, the moment they bump into a lithium atom, they grab onto it, and they sink to the bottom of the solution in one version of the machine. So it allows you to extract minerals from the environment that are not metallurgically available today.
For example, you know, I'm here in Palo Alto and the dirt in Palo Alto probably has enough iron in it to make the frame of hundreds of thousands, if not a million cars, but there's no technology on Earth today that can take a mound of dirt, put it into something and get a iron brick out. That's not really how current technology works. We have to go for high-grade ores to get iron bricks out.
If our technology works and if we're able to deploy it, you'll be able to unlock this kind of abundance. Now we're not setting up iron, because you know, it's a particularly abundant metal, but lithium, cobalt, rare-earth elements, titanium? These are the kinds of things that we'll target and ideally make highly abundant.
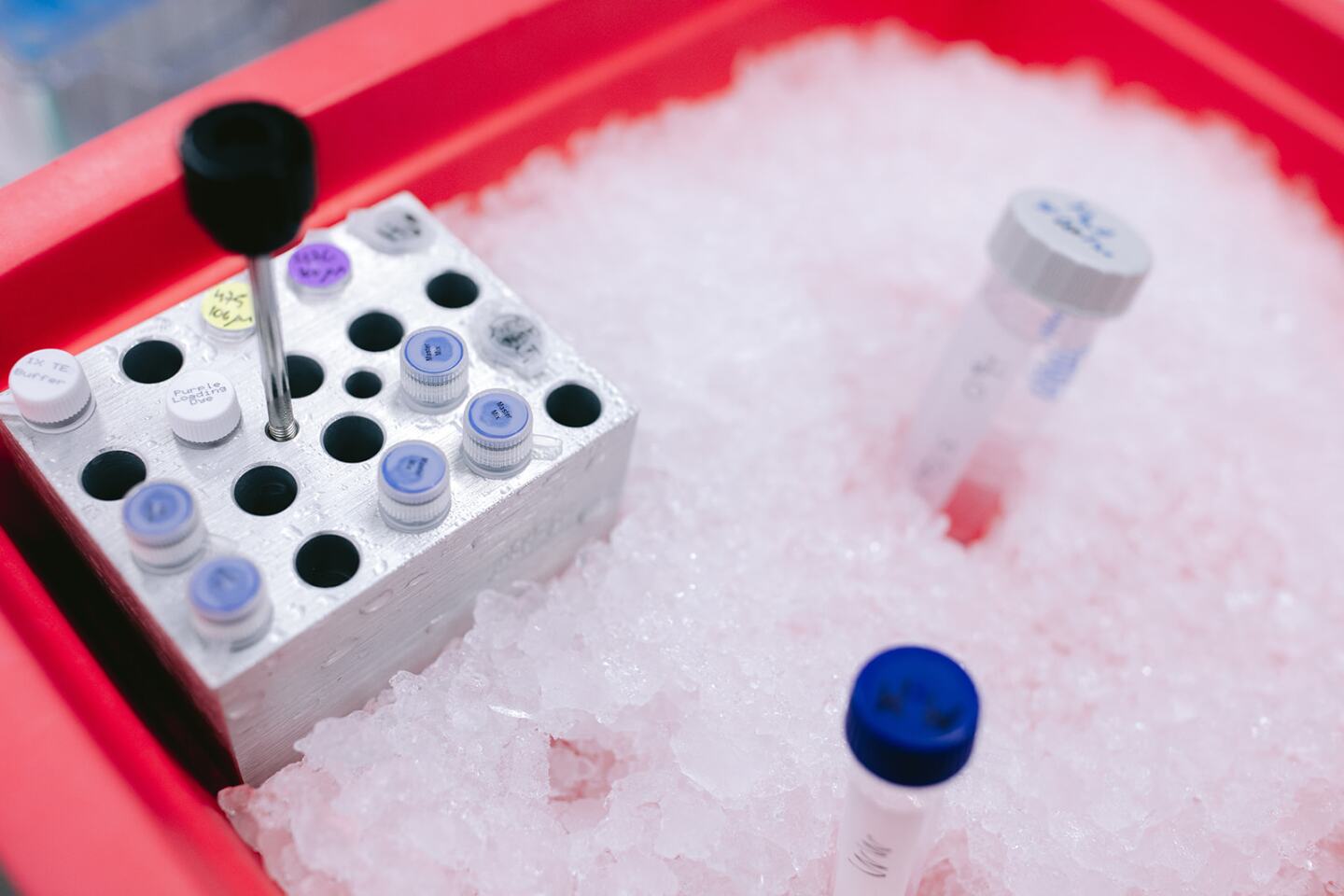
Right, so you have created this enzyme that will that will grab lithium out of seawater or brine. Was that a random find? Or was that something you specifically targeted?
That was directed! It's a great question, though. So the way our product process works, is we always start with this idea – and it's a very intentional strategic decision – of only working on what we call novel chemistry. We'll only try to create things with some functionality that is impractical or infeasible chemically, and impractical or infeasible biologically.
If we were engineering an enzyme to catalyze a chemical reaction that's already known, or that someone else can do, then the bet that I'm making when I'm creating this product is that Aether is smarter than tens of thousands of chemists that work at DuPont, right? I'm not very comfortable making that bet. They're very smart people working there, and there's tens of thousands of them.
I would much prefer to be working on products that those guys may have dreamed of doing, but could never figure out how to do. And when we look at these products, we come across these chemistries in one of two ways. We can discover them accidentally – a good example is our PFAS degrading enzymes. We didn't discover the enzyme that degrades PFAS accidentally, but we did discover the chemistry accidentally while we were running a different program. We're like, wait, we can do that? What can we do with that? What kind of product could we create?
In the mining situation, we said well, we think lithium is probably the best starting point for our minerals program. And we had our machine learning circuit designed for some of these proteins that can bind to lithium, and let's see where we get. And we actually found some, which was very exciting.
Alright, so what's what's the path forward with that one? Do you guys become a lithium mining company? Do you license that technology?
Yeah, so the current strategy with lithium is we want to deploy an on-site pilot somewhere in the United States, say in the middle of next year if things continue to go up, which is always harder than I want it to be. So perhaps a little later than that.
And once we prove the technology out in the field, we would probably become a lithium supplier, or at least part of our company would become a lithium supplier, and then we'd start to stack additional metals on top of that. Because the thing that's quite exciting about the minerals component of our company, is the approach and the technology is somewhat modular, not 100% modular, but reasonably so.
So for example, if we were to have a shipping container-sized system that has a filter bed full of our proteins that bind to lithium, for example, and we're running some brine through it, and let's say we decide, well, we'd also like to extract cobalt from that stream of water? All we need to do is swap out the the nano-proteins that bind to lithium for ones that bind to cobalt. And for the most part, the rest of the process is the same. I mean, a little bit on the back end changes obviously, depending on the metal and how you store it.
But that level of modularity means that as we learn how to scale up and build commercial versions of our lithium plant, we're also learning how to build our titanium plants, our cobalt plants, our rare-earth plants. We really want to build that out, especially in the United States.
On the material side, we're also probably going to directly sell some of our polymers to begin with, and then, on a case by case basis, you know, sometimes it might make more sense to license some stuff out. For example, we have discovered some chemistry that can be quite valuable in pharmaceutical development. We're not a pharmaceutical company. So at some point, you might choose to license out those chemistries to a pharmaceutical company and say, hey, why don't you guys go see if you can create a life-saving therapeutic. We'll focus on our areas that we specialize in.
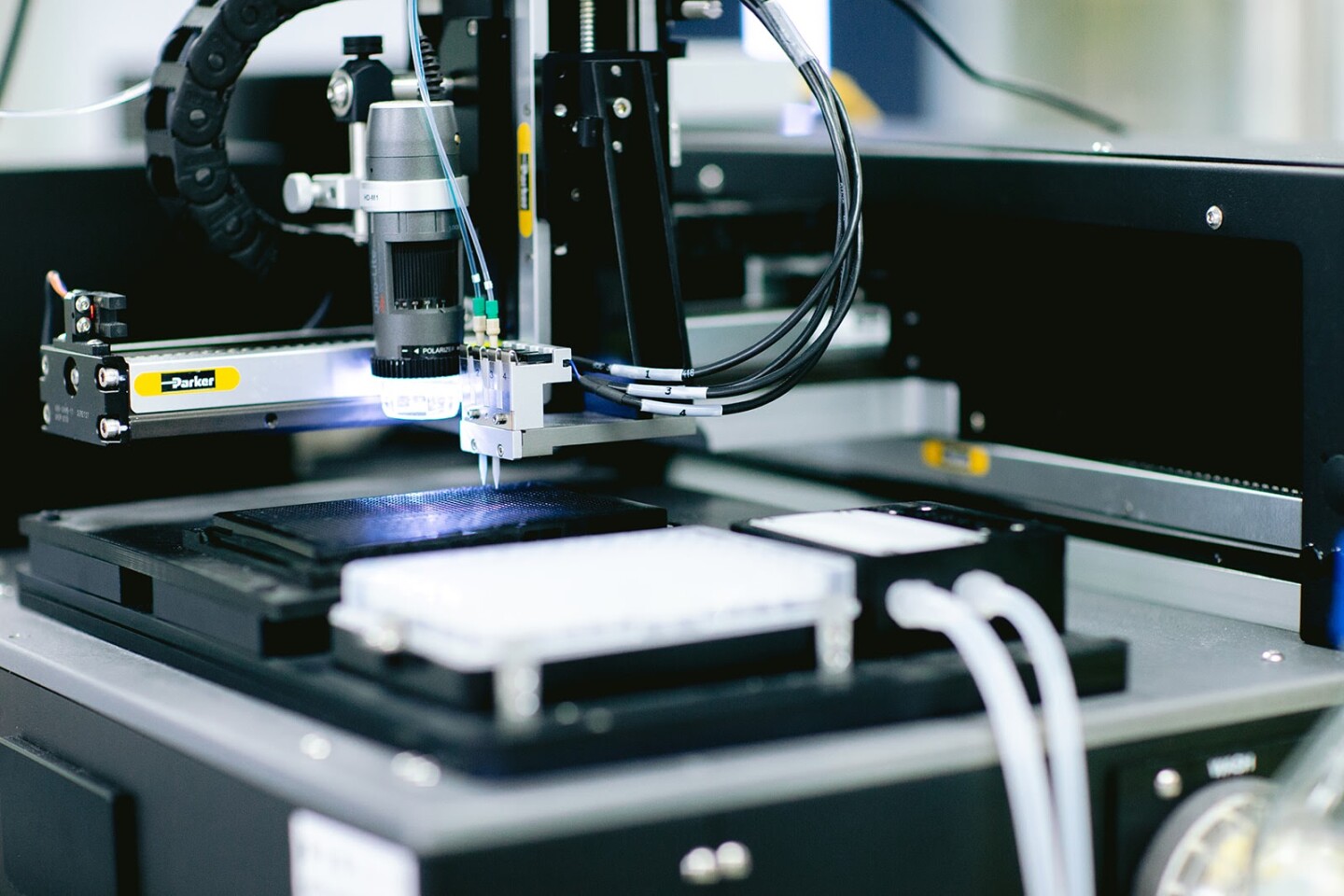
Okay, just on a more basic level, how complex are these molecules?
You know, they're not that complex. A lot of the nano-proteins we create, you're looking at few hundred amino acids and some additional stuff attached to them. That's not particularly complex. What's really unique about the machines that we engineer is that they usually look nothing like what exists in nature. And through that process, we've been able to create machines that have functionalities that just nobody thought were even possible. The smallest design that we're actively testing right now is only about 300 times heavier than a lithium atom.
Such a wide range, so many orders of magnitude. I think that if you extrapolate out five, 10 years from now, or more, assuming we survive, and we're successful, and all that kind of stuff... The kind of molecular machinery we're building, it's meant to have many of the incredible capabilities that you read about in science fiction, Arthur C Clarke and all that kind of stuff. What I'd call maybe true nanotechnology. But it won't look very much like that.
The machinery at the nanoscale operates on very different principles to machinery at our scale. I mean, there's a lot of things that are just very fundamentally different. Even something as simple as a gear would work very differently at the nano scale.
So right now we're not trying to work out how to build the full, complete nano-machines. We're gonna start working on that in a few years. Right now, we're just trying to figure out: how do we build the constituent parts? How do we get to the point where if I just need a nanoscale part that's made of this many atoms in this pattern? How do I just assemble that very, very quickly so I can test that part and see what it does?
And it turns out, there's some really wonderful ways to commercialize that through mineral extraction and new types of polymers that we're pursuing. But long term, you know, it's really about building those constituent parts for even more complex machines at the nano scale.
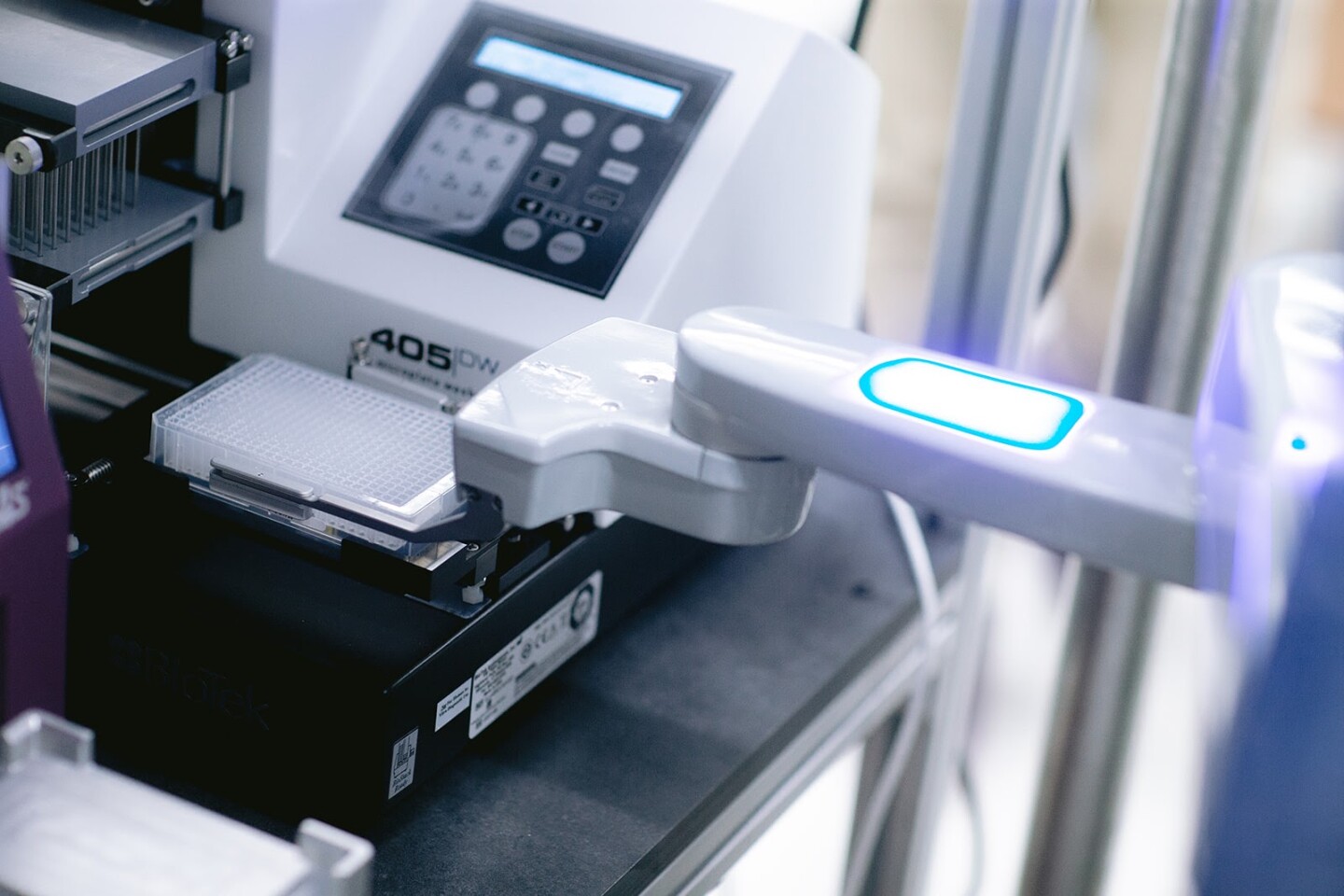
So, in a very basic sense, how do you assemble these little machines?
It's fairly simple actually. Most of these machines, they start off looking a lot like proteins. So the initial part of our process is we take DNA that we've custom ordered or modified or synthesized ourselves, and that DNA codes for whatever this kind of protein building block core is going to be. We go through an automated process, we convert that DNA into protein. And we've developed a lot of techniques to do that really effectively, quickly and at low cost. And then depending on which program we're working on, we will chemically modify these machines even further.
For example, a lot of our mineral-binding nano-proteins, if you want to call them that, they're not only different than normal proteins in that there's nothing that looks like this in nature, but we actually... You know, typical proteins are linear. So there's a beginning and end to this chain that folds up into a structure. We actually connect them into rings, and even into sets of rings.
And we do that because we basically create these threads of these little proteins attached to each other. And as lithium wafts by, it's almost like a net. Water is allowed to go through them. But when lithium goes through, it just latches on, it doesn't allow it to move any further. Actually, a fishing net is a reasonably good analogy of what how it works at the nano scale. So we always start with something that, at least in principle, could be called a protein, but then we sort of go beyond that and say, well, what are the modifications to this that we need to make it really efficient at scale?
That's remarkable. Obviously, you can't see these structures. How do you know what structures they're folding up into? Sorry if this is a first-year chem question!
We don't necessarily know the structure that they fold into, but we test their function. So you know, we can use software like AlphaFold 2, we certainly have access to all these kinds of things. But at the end of the day, what matters most is regardless of what the structure is, is it doing the function that we want? So we use a very high throughput laser mass spectrometry technique.
What we might do is say, well, we'd really like to find something that binds to lithium and only to lithium. We don't want to bind to magnesium, or calcium, or iron or any of these other things. So we ask the machine learning, can you please design you know, a thousand versions of this machine?
We synthesize them, and then we mix them in a solution that has lithium, magnesium, calcium, and iron. We let that dry out. We hit it with the laser and we see whether all of our machines are bound to lithium, or are some of them bound to magnesium, or some of them to calcium? And through that, we're able to say, hey, 96% of these are bound to lithium. That's great. For another design, maybe only 2% are bound to lithium and it's mostly bound to magnesium – so we don't keep engineering that one.
Machine learning trains off that data and we sort of rinse and repeat, rinse and repeat. So it's very much an automated experimentation process, where typically, the structure is what we would expect it to be if we simulate what the structure should look like. But we kind of don't care, you know, as long as it does what we want it to do. That's really what matters and if the structure is a little bit different, you know, fine.
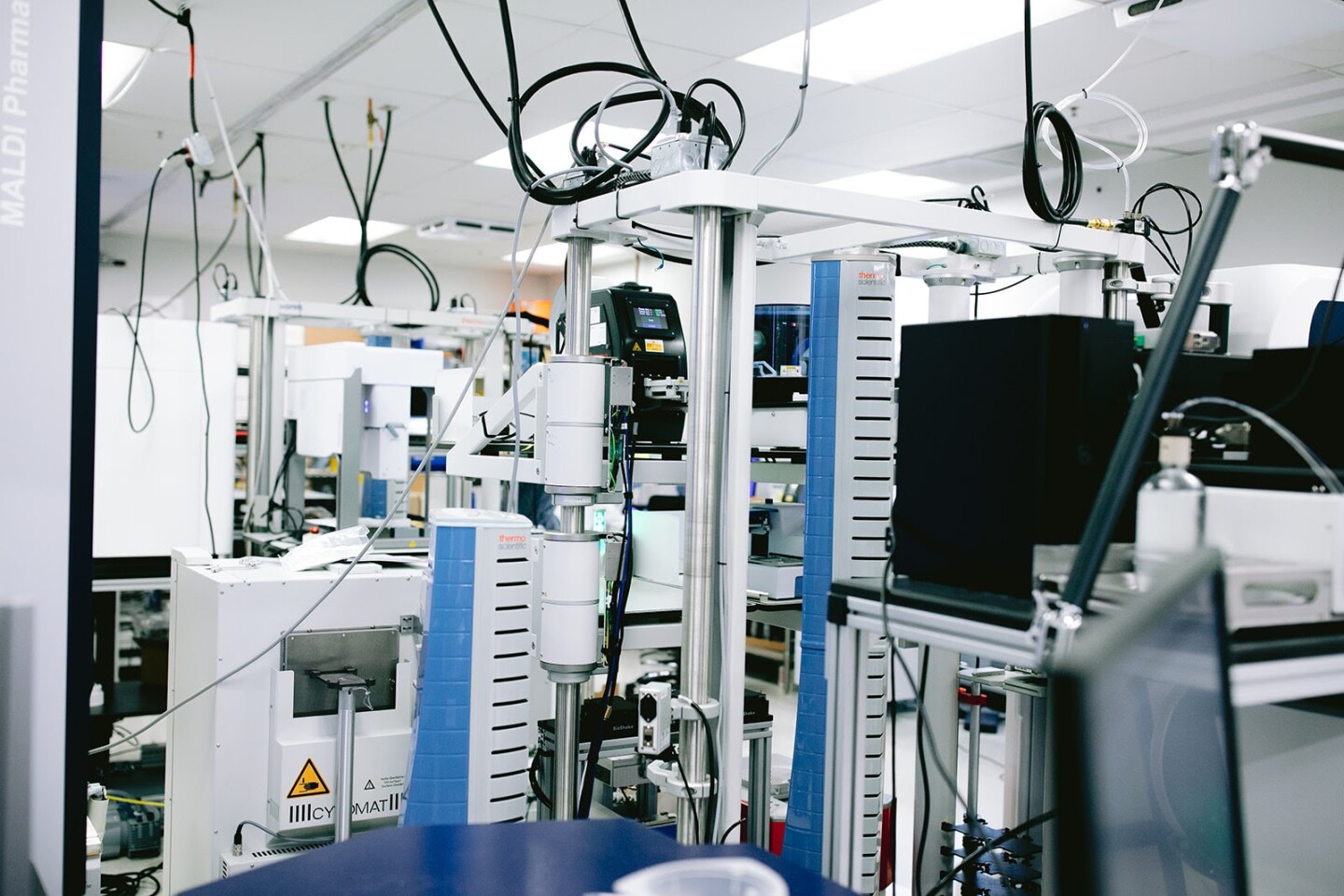
Fair enough. So mineral extraction as an early case. What are your eventual dreams for this stuff?
One of the main milestones for the company, from a long-term vision perspective, is to achieve what I currently call total molecular prototyping. I'm sure I can come up with a better name given a little more time. But this is the idea that we get to a point where that sort of input-output arrangement of atoms is a done deal. We know how to do it. We can engineer one of these machines to rearrange the atoms programmatically. We can do it within a very short period of time, with a very high success rate. Today it still takes months for us to engineer these things and we can't do too many of them in parallel. Ideally, we get to a point where it's a foregone conclusion that it's going to work.
I guess the more you've made, the quicker you can target things.
Exactly. So we're building up these assets. We're getting better at it. We're learning what works, what doesn't work. And so we're getting faster, and it'll take probably another two to three years before we're really damn fast to setup. Once you reach that point, though, the mission of the company is to build a future of abundance for the human race. If I just get to this first milestone, all I've done is get really good at making fancy molecules that generate a lot of revenue. I haven't built a future of abundance. So how do you go to that second step?
Well, there's a concept in chemical manufacturing called continuous chemical manufacturing. And all it means is, rather than having a massive reactor that you sort of run a chemical reaction in, you sort of do it continuously. So maybe a gallon a minute being continuously manufactured, rather than a million gallons being manufactured over a million minutes, right? It's almost like an assembly line to manufacture molecules.
This process is very rarely done today, but it's incredibly efficient when it's done properly. It allows you to have machines the size of shipping containers that can be as productive as massive chemical plants, but in the size and the cost of something that can fit in a shipping container.
And the reason why this technique isn't often used today is because traditional chemistry is not really designed to run continuously, right? When you're doing chemical manufacturing today, it's not uncommon to have one step at 400 degrees Celsius, and one step at minus 20 degrees Celsius. And you think about that, if you try to run that continuously, that's almost absurd. You'd have to cool it down so fast, it'd require an enormous heat sink, just to make that happen.
So if we can achieve this total molecular prototyping in the next, let's say, three or four years, we've reached a point where we can make all these chemical reactions happen under basically the same set of conditions or at least roughly room temperature and roughly the same set of conditions. So we don't have that limitation anymore.
That means Aether as a company can start to build shipping container-sized systems – not just that can extract metals, but that can also take very cheaply abundant starting materials that are available in different parts of the planet, and assemble them into very complex pharmaceuticals, materials or what have you – without having spent billions of dollars building gigantic factories.
So I think, if we want to create a world where 10 billion-plus human beings live and consume at a rate that you or I might consume every day, the Earth can't support our rate of consumption right now. And it's not like all seven or eight billion of us are consuming at this rate yet.
I think you need to create a new form of industrial manufacturing where it's unbelievably scalable. It operates out of shipping container-sized systems that are just spread all over the planet. It's decentralized. And it can create products that we just simply can't think of today. It really enables you to freely design molecules and arrangements of atoms you couldn't have thought of before. So that's really what the long term plan is.
Part of that is, how do we get really good at making just about every core atom cheaply available? That's one of them. Two: how do we get really good at rearranging those atoms in programmatic ways? And three, how do we get from creating complex molecules to creating even larger three-dimensional structures with these machines? We're working on one and two right now. Number three will be very difficult, certainly, to actually create three-dimensional structures using these machines, but it should be possible.
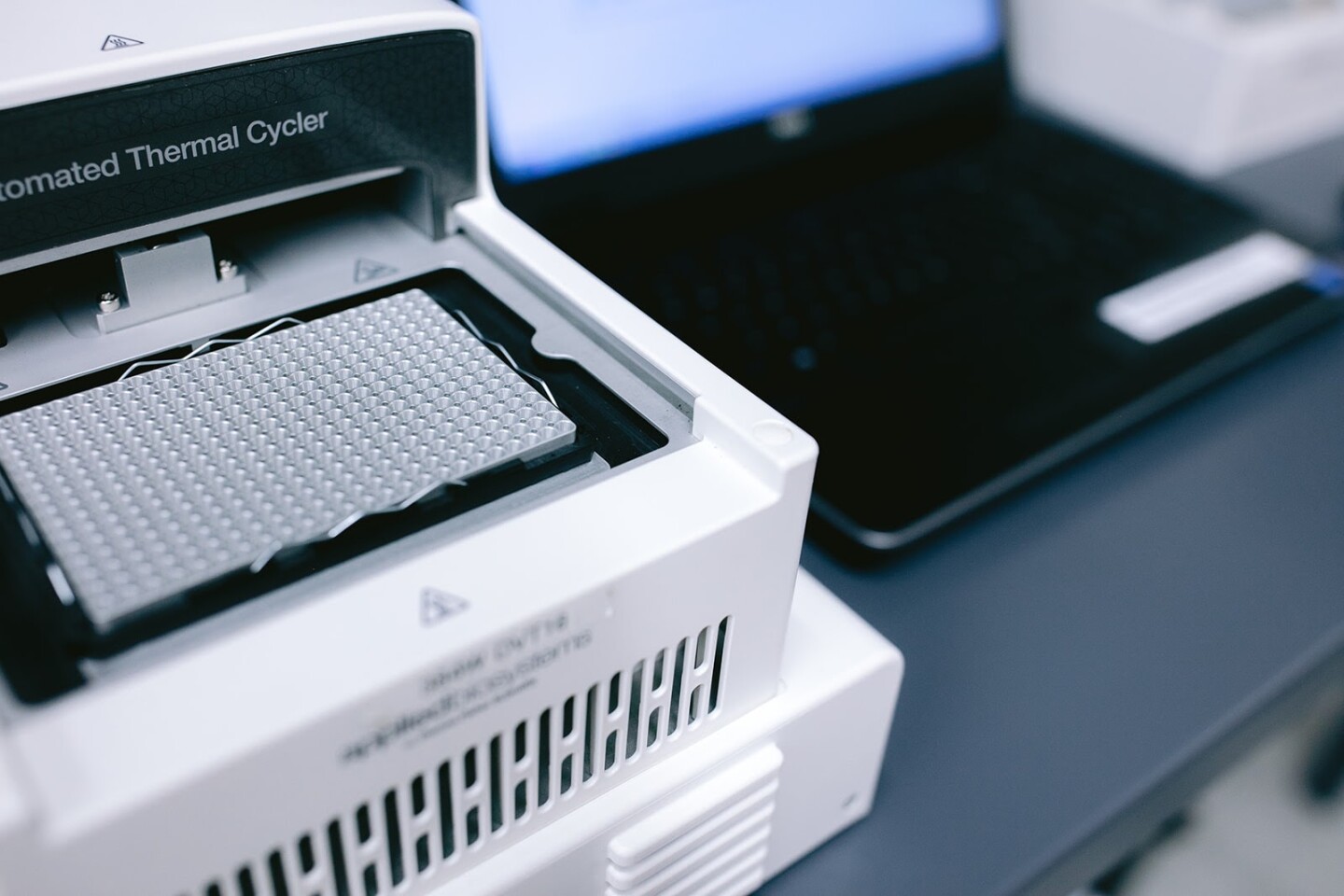
Right. So how long have you been going for thus far?
About six years now. Yes, it's been a while.
Okay. And what have been the milestone achievements in that time?
So we proved out our technology. We succeeded at a pharmaceutical contract. We were able to discover the first PFAS-degrading enzymes in our partnership with Allonnia, which was quite exciting. And then yeah, we've been focused on the lithium materials program since then.
So with the PFAS-degrading enzymes, that's the old "forever plastic." Given that these microplastics are now absolutely everywhere, how could that enzyme be practically used, like as a water filter?
Exactly, it's a water filter. And actually, this is a really good example. So you asked earlier, do we license or do we go to market ourselves? So we sometimes find a chemistry that we think would be very valuable, and also be helpful for the human race, but it's not directly aligned with our mission.
Water purification is a good example. We think there's a ton of value. But Aether isn't a water purification company, we want to be a manufacturing company. So when we discovered this new chemistry, we partnered with another company to license the technology. This will be valuable to someone, it will help human health. But it's not us. This is not what we're gonna do. And the same thing is true with pharma. We have to be focused on something, otherwise we'll never get anywhere.
Okay. So you're looking at the resource extraction as a way to finance the more exotic stuff?
Exactly. There will certainly be high-margin materials we can sell. On the metal side, if our calculations and our modeling are correct, we'll be very high-margin on that front, just because we can extract some of these metals at incredibly low cost.
Just as an example, imagine what we could do if titanium was as cheap steel. The kinds of things that become possible from an engineering perspective are pretty incredible, not just in automotive... Buildings could be constructed out of titanium.
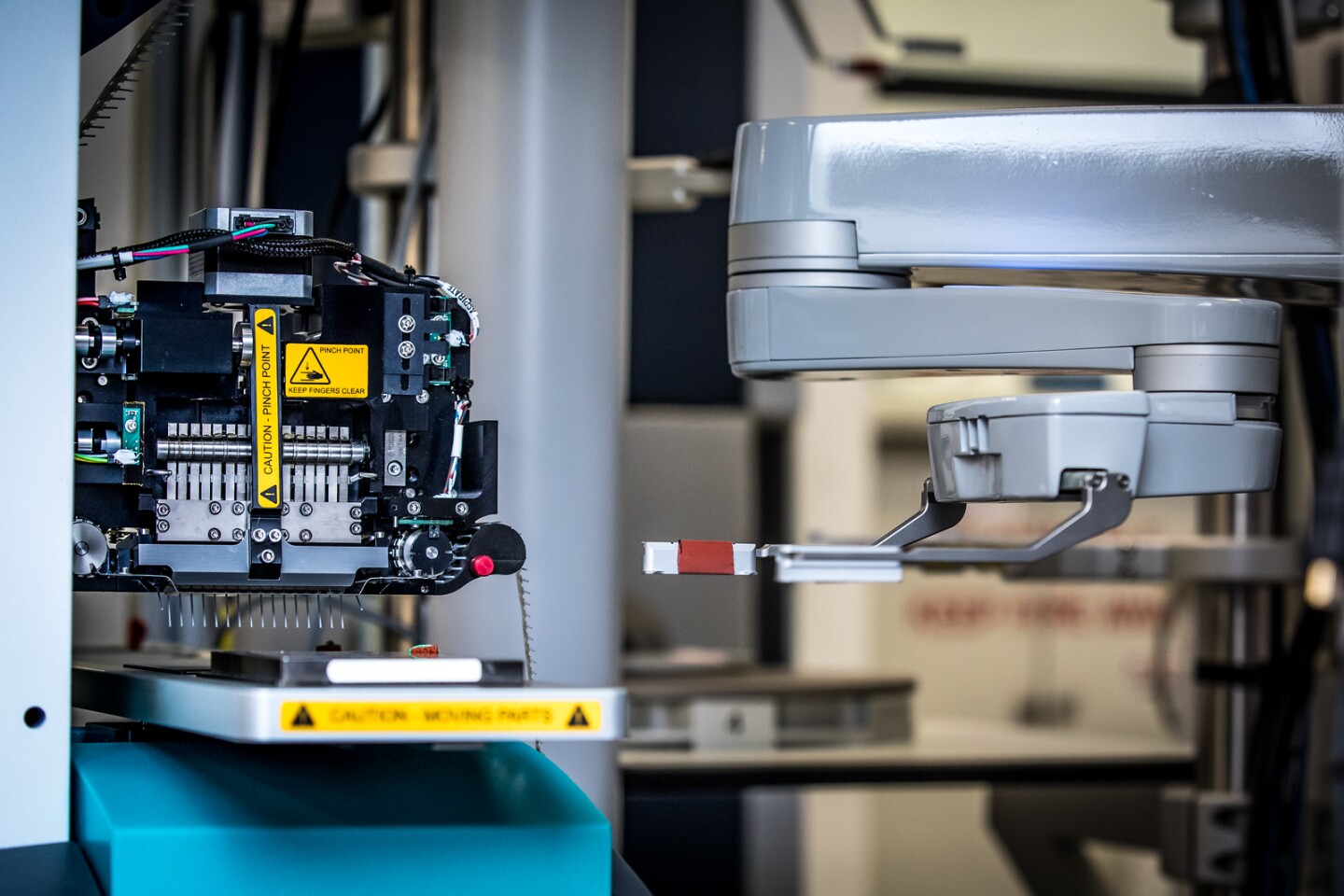
So with lithium, that's found in seawater and in certain underground brines. With titanium, where does that exist naturally?
There's a few forms it takes in nature. There are some forms that are water soluble, usually the titanium salts, and then there are some forms titanium that are not soluble in water – the hydroxides. There are water tables that have titanium salts in them, in substantial amounts. They're just too low of a concentration for anyone else to access.
It's similar to lithium, everyone and their mother is trying to extract lithium out of the Salton Sea. And they're doing that in part because the concentration of lithium in the Salton Sea is some of the highest in the United States, you know, 500, 600, 700 parts per million.
But we strongly suspect that the vast majority of the lithium in the world is actually at lower concentrations, 100 to 250 parts per million. Seawater is actually much less, we won't tackle seawater. Even if I can extract that 50 parts per million lithium instead of 500 parts per million, I have to pump 10 times the amount of water to extract that lithium. So at some point, it's like okay, that's just too much pumping.
But there are quite a few aquifers. And there's not just aquifers – there's also produced water, which is a waste product of oil production today in the United States. And there are huge fields of oil derricks across the United States, where they're no longer operated and producing oil because they hit the water table. Well, that water has metals in it. So we can imagine in some cases, us converting spent oil fields into lithium fields.
Do you guys share an estimated cost that you might be able to recover lithium at?
Yeah. So in the current version of the system that we're designing, we would be cost-competitive with people trying to extract lithium at 500 parts per million, but at 100 parts per million. And if we were operating with a 500 parts per million brine for our source, we would be about 60 to 70% cheaper than anyone else.
So in terms of quick numbers, as far as we can tell, most people that are claiming to be able to extract lithium from the Salton Sea are at about US$4,500 per ton. For us, at least in the system were designing, we'd probably be at a little below $2,000 per ton. We'll get dramatically better, closer to $1,000 per ton over time. And working with lower concentrations, say at 100 parts per million, which no one else can economically access today, we're at about $4,000 per ton.
Okay, so you wouldn't have to compete with anyone else for locations.
That's exactly why it's exciting to us. These sites are not useful to anyone else. And the vast majority of lithium is there.
Very cool. So you guys have just done a raise.
Yeah. $49 million.
What's your total funding level at this point?
Just north of $60 million.
Okay, and that takes you through to the point where you should be producing your own lithium?
Basically the purpose of this series A is to deploy our pilot systems out in the field. And it's really about validating – hey, we found something interesting in the lab, now to show that it actually works out by an oilfield, or via brine sample, or that we can manufacture these materials at scale, and then signing the supply contracts and then we go raise our series B.
Hmm. So the mass manufacture part of it, what are the challenges there?
They're different for both areas. So on the materials side, the manufacturing is actually relatively straightforward for these materials, which is great. I'm glad it worked out that way. It's really more the formulation, and developing the final product that's quite challenging. How do you blend it? How do you melt it, all that kind of stuff. We're dealing with a new class of polymer here that really hasn't been worked with before. So that's where I think the challenge is going to be.
And the opposite is true on the lithium product. So we know what to do with the lithium. Once we have it, we know how to sell it, we know how to convert it to battery-grade. That's a well established process, but no one's ever been able to extract from these lower concentrations.
So we're very much focused on de-risking for lithium as quickly as possible. So start building larger and larger systems. We're getting samples of brines from across the United States and actually from across the world, and testing our system on those brines. And on the materials side, we're much more focused on, how do we make sure that the product has exactly the operating effect, and that it's properly formulated and it's stable for a long period of time, all that kind of stuff.
So after you gather a certain amount of lithium, you just replenish the enzymes?
Yeah, exactly.
How do you get the lithium back out?
There's a few different ways you can do it. The easiest way, which is actually close to what everyone else does, is to run water through it. So from the start, you basically take your filter that is full of your nano-proteins, and you run your brine through it, and as it runs through, the lithium gets caught in that fishnet of all these little machines. After maybe an hour, it becomes saturated. So you shut your brine off.
And one of the cool things about these little nano-proteins is we could engineer them to bind to lithium in one condition, but then to sort of release the lithium when they see a different liquid. The system we'll probably deploy at scale won't use water to wash out the lithium, but it depends on the location. If there's a lot of water available, we might use that.
But we'll probably use a different solvent entirely. I can't... We haven't filed the patent yet, but you can basically imagine that in the presence of this solvent, they're programmed to basically release like a spring and just shoot off the lithium and it just floats out. And then you get your lithium on the back end, and concentrate it, and then you have battery-grade lithium.
So it's not just that you can access lower concentration lithium than what anyone else can. You also don't have to pre-treat your lithium stream, which is what a lot of other companies have to do. And in a lot of versions of our system, you get directly to battery-grade lithium on the back end, which is unprecedented. Today, you mine lithium and then you send it somewhere else to be refined into battery-grade. Our process, if it works, we think it'll just output battery-grade lithium that can be directly shipped to a battery manufacturer.
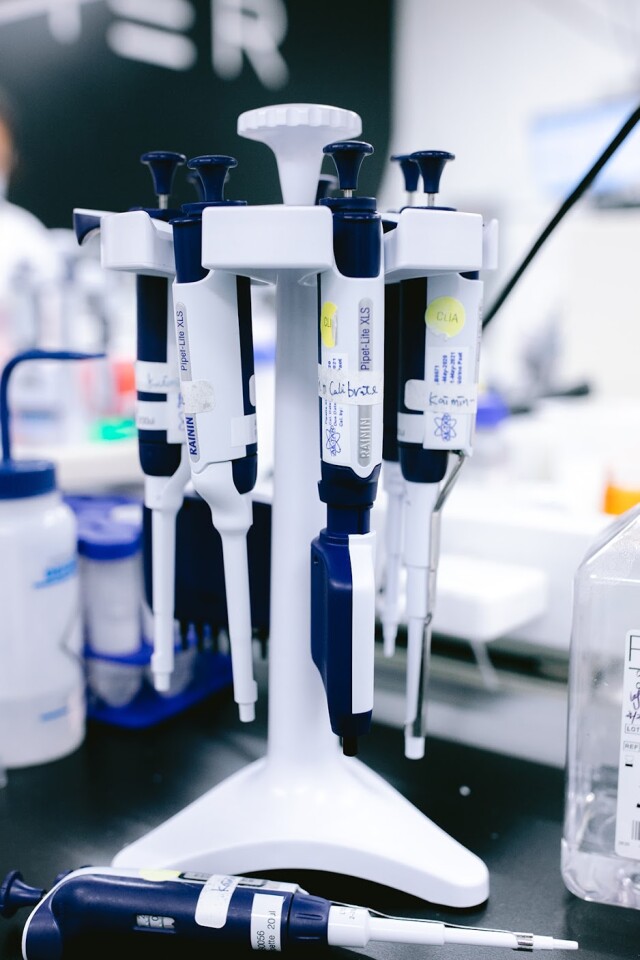
That's pretty wild.
And it's really because these little proteins, they don't bind to anything but lithium. So there's no refining necessary. It's just kind of pure lithium coming out the back end.
So for the case of a disused oil well, you're talking about a shipping container-sized unit?
Well, it depends on how much volume you're processing. I think the commercial demo system we're thinking about is probably the size of about five shipping containers. And actually, when I say shipping containers, it's not just as a unit of measurement. We're actually thinking about deploying in shipping containers.
When you think about the complexity of building any sort of factory, the moment you have to pour concrete and build a custom system, the cost and time explodes. But if we can build a semi-modular system that's just, you know, five shipping containers that are kind of like Lego, so you can stack them together in different configurations? It'll not only be cheaper for us, but I think we'll be able to scale much faster.
Hmm, yeah, you can just centralize manufacturing of those containers and fire 'em out.
Exactly, you build a giant factory that just makes these things and outputs shipping containers then just get set up across the country.
Very cool. In terms of safety of this stuff, you know, the "grey goo" nanobots scenario, you're kind of heading towards that kind of thing.
The very nice thing about the machines so far is that they are compostable. It's actually kind of a problem for us – bacteria love to eat them.
Okay. So not a problem at this stage. But I guess you're looking at getting towards the stage where if some of these things escaped, they might start eating things that they weren't supposed to eat. Do you have protocols around that? Or is it too early to be worrying about?
It's a bit early right now. But we do keep this in mind when we're engineering our machines. And we try to make sure that there's always a failsafe. These machines are designed to operate in a very limited set of conditions, and they're designed so that they don't work outside of those conditions. So for example, you take our lithium-binding machine and you just spread it across a cornfield. Not only are bacteria going to eat it very quickly, but it's just not going to work. The cornfield's not the right pH, it's not the right temperature.
So we very intentionally try to tune the machines so that yeah, it's a very narrow window in which they'll work at all, and they just break when they leave that window. That's partially for safety reasons, but honestly, it's also partially because it protects us from someone trying to figure out how our machines work. If you try to open the machine and scoop some out, they'll all be broken by the time you get into your lab.
Very neat, this is really cool stuff. Very out there. The potential here, obviously, is almost unlimited. So all the best. Good luck with it!
Thanks, it's been an excellent conversation.
Many thanks to Pavle Jeremic , as well as John Vollmer and Andrea Ambriz-Alvarez at VSC, for their assistance with this piece.
Source: Aether