In an apparent contradiction to textbook physics, a metal has been identified that conducts electricity but produces almost no heat in the process. Such a strange property may be expected to occur in conductors operating at cryogenic temperatures, but a team of researchers led by the Lawrence Berkeley National Laboratory claims to have discovered this unique property in vanadium dioxide at temperatures of around 67 °C (153 °F).
Of all the metals found on Earth, most are both good conductors of heat and electricity. This is because classic physics dictates that their electrons are responsible for both the movement of electrical current and the transfer of heat. This correlation between electrical and thermal conductivity is dictated by the Wiedemann-Franz Law, which basically says that metals that conduct electricity well are also good conductors of heat.
However, metallic vanadium dioxide (VO2) seems to be different. When the researchers passed an electrical current through nanoscale rods of single-crystal VO2, and thermal conductivity was measured, the heat produced by electron movement was actually ten times less than that predicted by calculations of the Wiedemann-Franz Law.
"This was a totally unexpected finding," said Professor Junqiao Wu, a physicist at Berkeley Lab's Materials Sciences Division. "It shows a drastic breakdown of a textbook law that has been known to be robust for conventional conductors. This discovery is of fundamental importance for understanding the basic electronic behavior of novel conductors."
And what a novel conductor VO2 is.
When heated to 67 °C (153 °F), vanadium dioxide undergoes an abrupt transition from an insulator to a conductor, as its crystal structure transforms. This structural alignment of VO2 into a metal provides clues as to why the material is able to transfer electrical current with negligible heating.
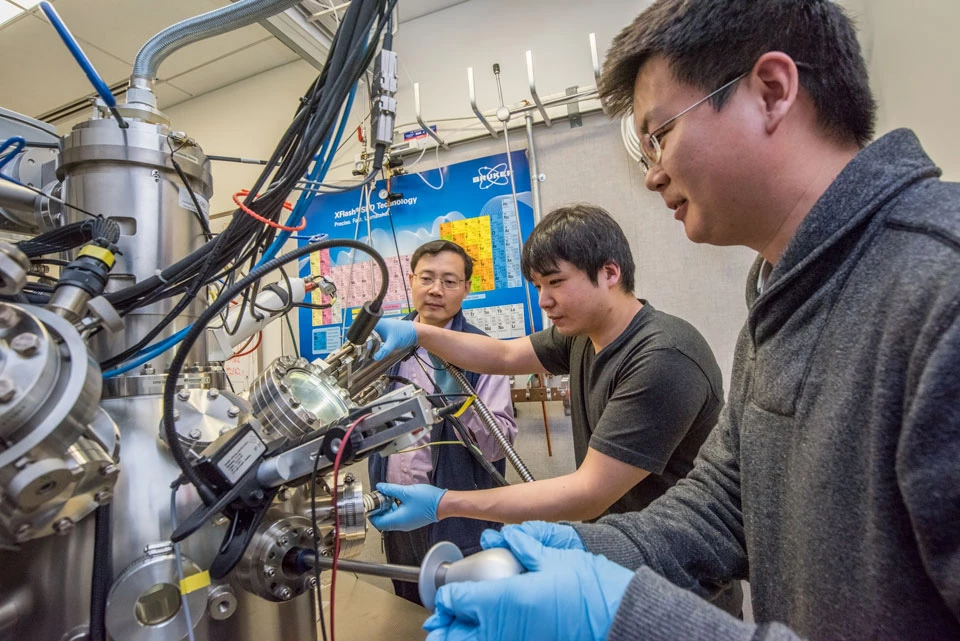
At the nanoscale level, the classical models for the conduction of heat begin to fail. As a result, other theories used in semiconductors, insulators and transient materials like VO2, are used where heat is seen to be carried primarily by vibrations in the crystal lattice known as phonons (originally from the ancient Greek, phonos, meaning "sound," but generally referring to any quantum-level compression wave).
At ordinary temperatures, waves of phonons traveling through metals can interfere with the ability of electrons to pass freely through the structure, because the phonons pitch atoms out of their equilibrium state, in effect making them roadblocks.
Conversely, at ultra-low temperatures, the vibrational waves of phonons are severely curtailed as atoms are almost "frozen" into immobility. This allows the free flow of electrons with almost no temperature rise, because the electrons are largely unimpeded so that they don't crash into each other and lose energy. This is the reason why many metals become "superconductors" at cryogenic temperatures.
However, when vanadium dioxide is transitioning from an insulator to a conductor, it also shows a remarkably low thermal energy release, indicating that electrons in the material are somehow able to flow freely to produce a current, but without the impediments to their movement found in ordinary metals that produce waste heat.
As a result of interpreting results from computer simulations, much mathematical modelling, and the observation of X-ray scattering tests, the researchers believe that this is because VO2 in its transition phase can be seen as a so-called "non-Fermi liquid."
A smooth versus a bumpy ride for electrons
The Fermi liquid theory is a model of the normal state of most metals at low temperatures, where electrons collect other electrons on the path through the material and, therefore, accumulate a mass as long-life "quasiparticles" (discrete packets of energy with momentum and position). As such, these quasiparticles are subject to high dissipation (scattering) rates in normal metals at room temperatures due to interference from surrounding particles as they try to flow through the material.
Non-Fermi liquids, on the other hand, are thought not to produce long-lived quasiparticles for the transport of charge and heat. In these materials, if the electron density is not in equilibrium, electron diffusion moves like a liquid from areas of high-electron-density regions to low-density regions, generating current as it does so. In these cases – and likely in the case of VO2 – the heat transfer is taken over by the phonons, where phonon-electron coupling carries away and dissipates heat as mechanical (vibrational) energy over the entire surface of the material.
Double duty
In other words, vanadium dioxide in its transition state appears to be doing double-duty acting partly as a conductive path, and somewhat like an insulator, to create a semiconductor-like state that keeps electrons in line and moving them forward in a uniform fashion.
"The electrons were moving in unison with each other, much like a fluid, instead of as individual particles like in normal metals," said Professor Wu. "For electrons, heat is a random motion. Normal metals transport heat efficiently because there are so many different possible microscopic configurations that the individual electrons can jump between. In contrast, the coordinated, marching-band-like motion of electrons in vanadium dioxide is detrimental to heat transfer as there are fewer configurations available for the electrons to hop randomly between."
In essence, normal Fermi liquid type metals have too many opportunities for electrons (as quasiparticles) to be knocked off track as they flow through, leading to the production of heat. As a non-Fermi liquid – such as VO2 likely is in its transition state – eschews the creation of quasiparticles, the electrons remain flowing in more or less a straight line without colliding into other electrons and dissipating heat in the process.
Morphing material
According to the researchers, vanadium dioxide is also able to have its electricity to heat ratios altered by alloying it with other metals. Doping a single crystal of vanadium dioxide with tungsten, for example, the researchers decreased the temperature at which the phase transition from an insulator to a metal occurred, while improving the electron flow.
Vanadium dioxide is already a fascinating material that is transparent below about 30 °C (86 °F), and reflective of infrared light above 60 °C (140 °F). It also has the ability to become fully opaque in just 100 femtoseconds (a femtosecond is a quadrillionth of a second) when pulsed by a laser. Now, with added properties in its arsenal, the researchers believe that it may also have a role in areas of electrical thermal insulation.
Further experimentation, simulation, and debate will need to occur to determine whether or not vanadium dioxide is a non-Fermi liquid at its transition point (particularly one that seems to break a fundamental law of physics), but the research will be sure to continue at Berkeley Lab and other institutions to verify and replicate these results in search of a solid explanation for the phenomena observed.
The results of this research were recently published in the journal Science.
Source: Berkeley Lab