It sounds like science fiction to say there’s invisible, undetectable stuff all around us, and it doesn’t help that it has the spooky name of dark matter. But there’s plenty of evidence that this material is very real. So what exactly is dark matter? How do we know it’s there? And how are scientists looking for it?
Everything we see around us – from plants to planets, stones to stars, people to the Perseus galaxy cluster – is made of matter. But all of this only accounts for about 15 percent of the total matter in the universe. The overwhelming majority, that remaining 85 percent, is unaccounted for – and we call it dark matter.
This name isn’t describing what this strange stuff looks like – it earns that title because it doesn’t absorb, reflect or refract light, making it effectively invisible. And there’s nothing that can explain it in the Standard Model of particle physics, which remains our best theory on the universe.
There’s a huge worldwide effort to try to uncover what dark matter actually is, but the natural question it raises is: if we can’t see it, feel it, hear, smell or taste it, how do we know it exists at all?
The answer is gravity.
How do we know dark matter is there?
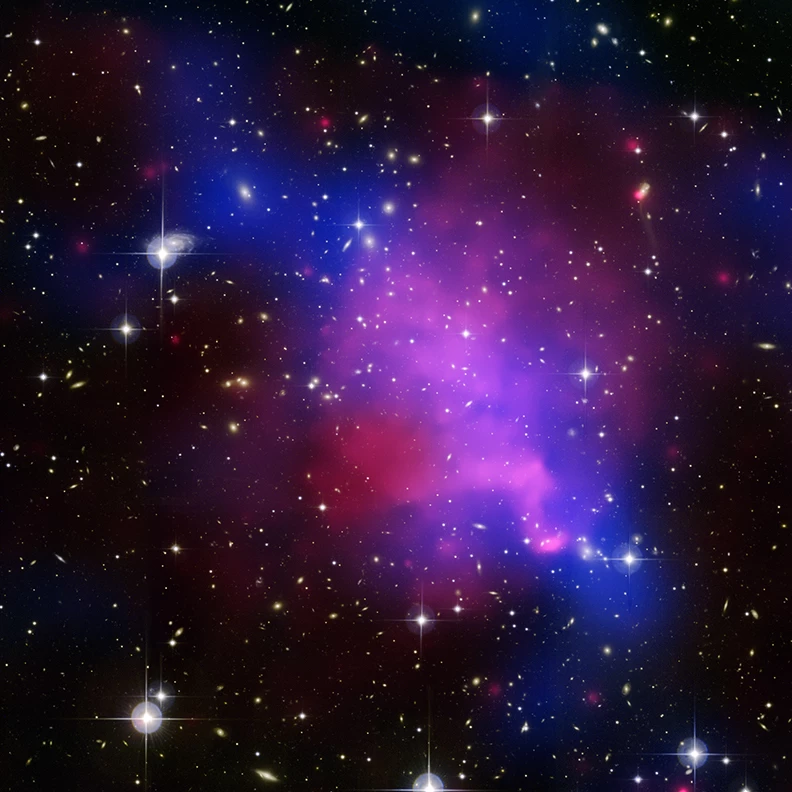
Anything that has mass has a gravitational pull, and the more mass something has, the stronger this force becomes. But astronomers consistently see that large-scale objects like galaxies and clusters behave like they have much more mass than what’s visible.
Swiss astrophysicist Fritz Zwicky was the first to propose the idea of dark matter in 1933. He was studying a cluster of galaxies and found a discrepancy: there didn’t seem to be anywhere near enough mass to account for how fast those galaxies were moving.
“When he looked at all the visible contributions to the mass that was in the cluster, he found that there was a huge shortfall in terms of providing the gravity that was needed to keep these relatively fast-moving galaxies from just leaving the cluster,” Raymond Volkas, Professor of Theoretical Particle Physics at the University of Melbourne, tells New Atlas. “Why was the cluster holding together at all? And so he surmised that there had to be another component that was gravitating but was invisible to us. And he called it dark matter.”
Zwicky’s discovery was just the first example of apparently missing mass. In the late 1970s, astronomers Vera Rubin and Kent Ford were observing our neighboring galaxy, Andromeda. The duo expected to see objects on the fringes of the galaxy orbiting more slowly than those closer to the center, but that wasn’t the case: instead, relative speeds tended to flatten off, with objects on the fringes orbiting far faster than the visible mass should allow.
Another strong piece of evidence is gravitational lensing. Since light beams are distorted by gravitational fields, huge masses can bend light passing by from more distant objects and make those objects appear larger or brighter, like a cosmic magnifying glass. Other times it can duplicate the image of an object, or even “replay” events like supernovae. Again, this lensing often occurs more strongly than should be possible from the visible mass of the object in the middle.
So we know dark matter is there. But it gets weirder – the universe as we know it couldn’t exist without dark matter.The Dark History of the Universe
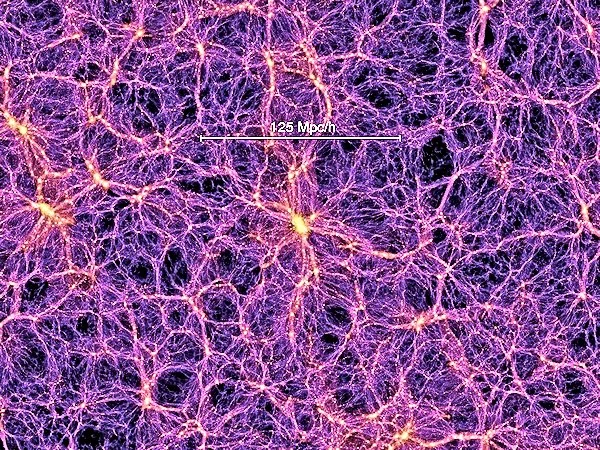
Just like the regular stuff, dark matter is believed to have been created in the Big Bang – or as one theory suggests, even before it, during a period of cosmological inflation. Either way, the structure we see out in the cosmos today would be very different without dark matter.
In the early days of the universe, everything was relatively smooth. We can see this today in the cosmic microwave background, which is radiation that was created some 400,000 years after the Big Bang. No matter which direction we look in, this radiation looks exactly the same.
But nowadays, the universe is far from smooth – it’s quite clumpy. These clumps are what we see as galaxies, clusters, superclusters, and other gigantic structures, and there’s always relatively empty space in between them. For example, right next door to the Milky Way is the “Local Void,” a region of unfathomable nothingness that spans hundreds of millions of light-years.
So how did the universe evolve from super smooth to clumpy clusters? That’s dark matter’s influence at work.
Even in the smooth early days of the universe, some regions had slightly more dark matter than others. This extra mass meant greater gravity, so these denser areas then attracted regular matter, which in turn attracted more and more. Eventually the heat and pressure caused these pockets of matter to ignite as stars, kickstarting the formation of the planetary systems, galaxies, and clusters that we see today.
The fact that the universe is structured the way it is is further evidence of dark matter. So we know it’s there. But what exactly is it? And how are scientists searching for it?
The hunt for dark matter
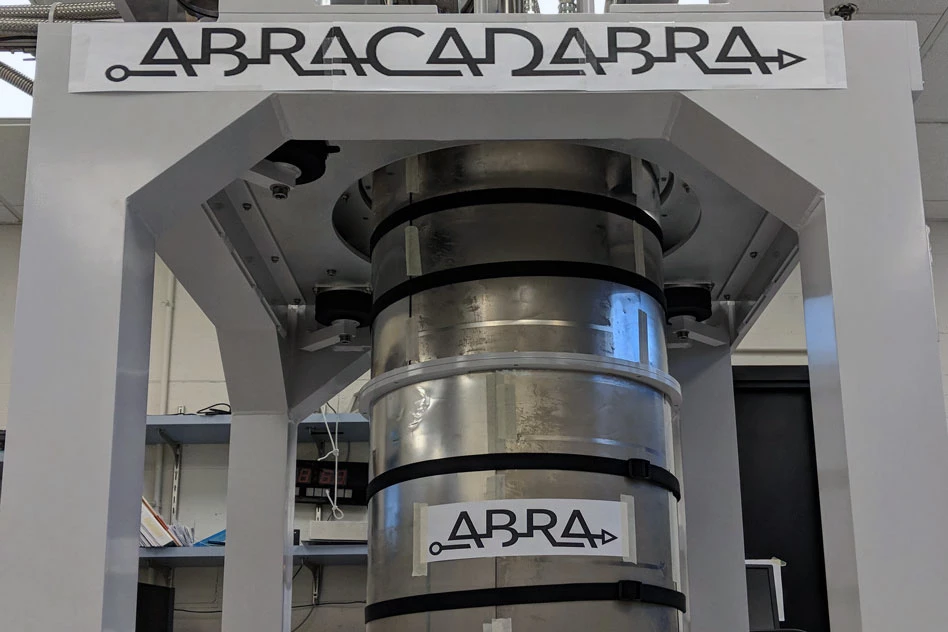
It’s not easy to look for something that’s invisible and rarely interacts with regular matter. So, scientists start by theorizing what dark matter could be, and then design and conduct experiments to test each hypothesis. The problem is, dark matter could be almost anything.
Dark matter particles could be among the lightest in the universe, or they could have the mass of a dwarf planet, or anywhere in between. Dark matter could be “hot” or “cold,” which has nothing to do with temperature but describes how fast it moves. It could exist in excited states, or have lower energies.
“Famously there’s an embarrassing plethora of different hypothetical particles or sets of particles that dark matter could be,” Volkas tells us. “And the range of masses and other properties for these dark matter candidates is enormous, so we simply don’t know.
“Theoreticians are very adept at coming up with speculations about what the dark matter could be, and most of them are very sensible speculations. So they could all be true in principle – but they won’t all be true at once. And so what we need to do are experiments and astronomical observations to try to narrow down the possibilities and come at the truth.”
Can CERN create dark matter?
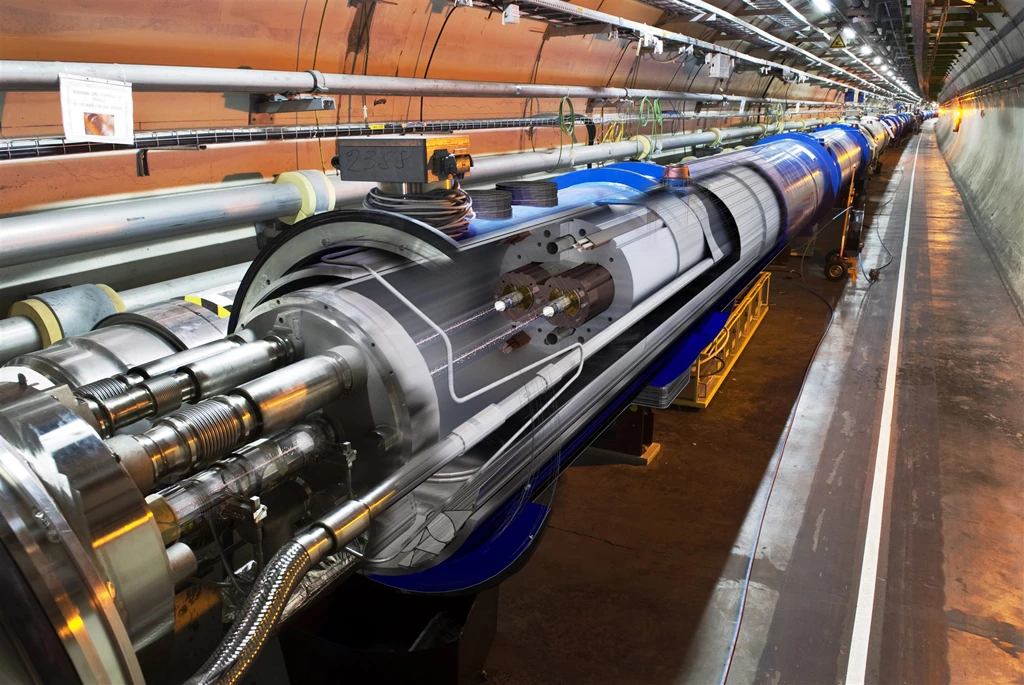
Different types of experiments are hunting for different theoretical dark matter particles. Perhaps the most famous experiments are those being conducted by CERN, at the Large Hadron Collider (LHC). There, scientists are looking for dark matter by trying to create it.
In the LHC, protons are made to collide at extremely high energies, producing a shower of other particles. Sometimes those are exotic particles that scientists normally wouldn’t have access to, and the hope is that dark matter may be among them.
Again though, if dark matter was produced in one of these collisions, it would be impossible to directly detect – instead it would just float off out of the tunnel without interacting with the detector. But that non-detection is exactly what scientists are looking for.
In physics, the laws of Conservation of Energy and Momentum state that in an isolated system, neither energy nor momentum can be created or destroyed. They may change form, but the amount will stay constant. So the scientists can calculate how much energy and momentum went in before the proton collision, and measure how much there is afterwards. If there’s anything missing, that suggests that something – like dark matter – escaped and carried away that energy or momentum.
Although the LHC has performed quadrillions of these collisions over the years, so far no suspicious dark matter signal has been spotted. But that helps to narrow down the wide range of possibilities, so future searches can be more focused.
Maybe the answer will finally come after the LHC’s High-Luminosity upgrade is completed in 2026.
Direct detection of dark matter
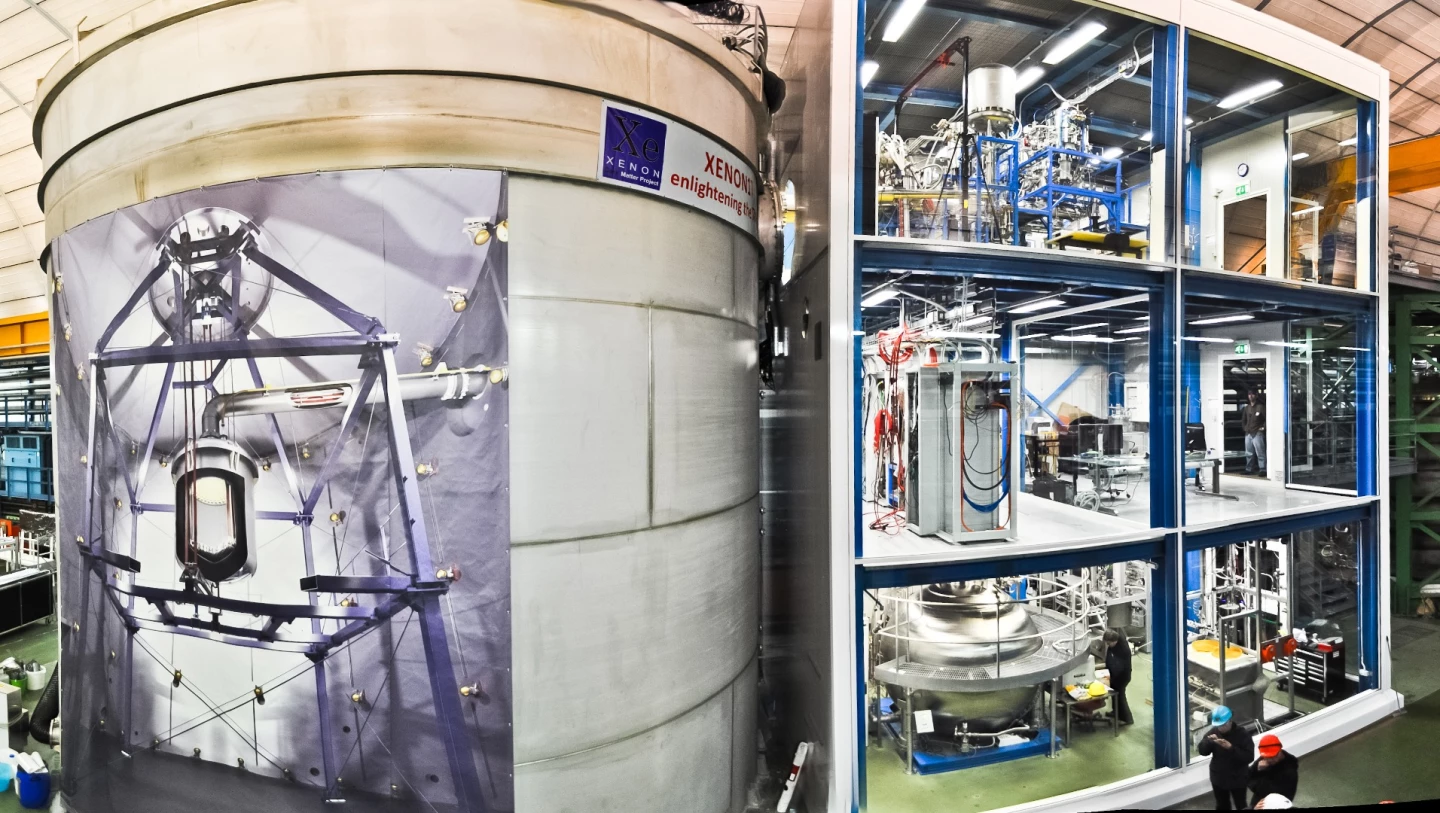
While the LHC is searching in one part of the spectrum of possibility, other experiments are trying to detect it in different ways. These studies are banking on the chance that dark matter may sometimes interact with regular matter through means other than gravity.
“The LHC is only sensitive to some kinds of dark matter,” Volkas says. “There are other sensible dark matter candidates for which the LHC is the wrong experiment. Another way to look for dark matter is through something called direct detection experiments. So the idea is you take a suitably large detector, you put it in a very quiet environment which is free from background influences that could mimic your dark matter signal, and then you just watch the detector and you wait for the nucleus of an atom to suddenly zip off for no apparent reason. The idea is that a dark matter particle has come along, hit the nucleus and caused it to zip off.”
This basic concept has been put into practice in various experiments all around the world. The detectors are usually placed in deep underground chambers, away from interference like cosmic rays or electromagnetic signals. And they’re all searching for different hypothetical dark matter particles, using different substances as the detector.
Experiments like LUX and XENON1T used huge tanks of xenon to try to detect a dark matter candidate known as a weakly-interacting massive particle (WIMP). The idea being that when these theoretical WIMPs bump into a xenon atom in the tank, they would give off a flash of light that instruments can detect.
Another proposal would use superfluid helium instead. The logic is that helium has a much lighter atomic nucleus than xenon, so it should be more sensitive to a bump from dark matter. That means it could pick up dark matter particles that are 10,000 times lighter than other experiments.
A variation on the idea is what’s been called a “snowball chamber.” This proposal uses a tank of pure water that’s supercooled to -20 ° C (-4 °F). At those subzero temperatures, the slightest disturbance to the water molecules can trigger flash freezing. So if it suddenly ices over for no apparent reason, that could be a dark matter signal. The advantage there is that water is far cheaper and easier to come by than xenon or superfluid helium.
Other scientists are tackling the problem in a completely different way. One proposed detector suggests using an array of a billion tiny pendulums, suspended in an extremely still environment. If a dark matter particle happens to whiz through the instrument, its gravitational influence should set a row of these pendulums swinging.
Missing in axion
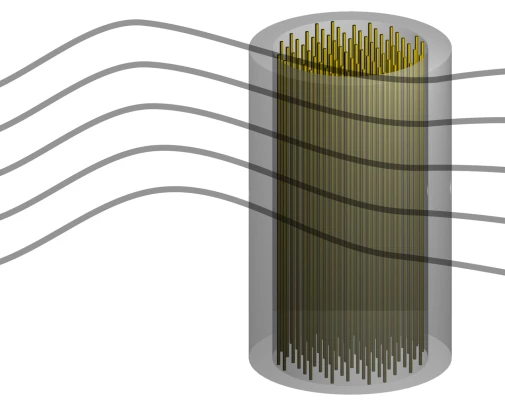
One of the leading dark matter candidates is a hypothetical particle called an axion. If they exist, these would be electrically neutral, very light, and drift around everywhere in waves. But most importantly, they should have tiny but detectable interactions with electricity and magnetism – and that just might be how they reveal themselves.
The ABRACADABRA experiment is designed to look for the magnetic fingerprint of axions. The idea is that because of how electromagnetic fields work, there should be no magnetic field in the very center of a ring-shaped magnet. So if you set one up and watch the middle, an axion could make itself known if a magnetic field spontaneously arises there.
In a similar idea, scientists at Stockholm University proposed a device they call an “axion radio.” The detector also uses a powerful magnet, but at the center is a chamber filled with cold plasma that contains a forest of ultrathin wires. This time, any axions passing through would produce a small electric field that would drive oscillations in the plasma.
The nEDM experiment is searching for axions in a different way. Here, neutrons are trapped and electrified, then their spin is monitored. The high voltage should affect their rate of spin at a certain frequency – and if that frequency is seen to vary over time, it could be a sign of axion interference.
In a similar experiment, physicists at Fermi Lab are hunting for axions using a supercooled, superconducting cavity containing a quantum bit (qubit) antenna. The idea there is that the magnetic field could convert passing dark matter particles into photons in the cavity, where the antenna can detect them.
Astronomical observations
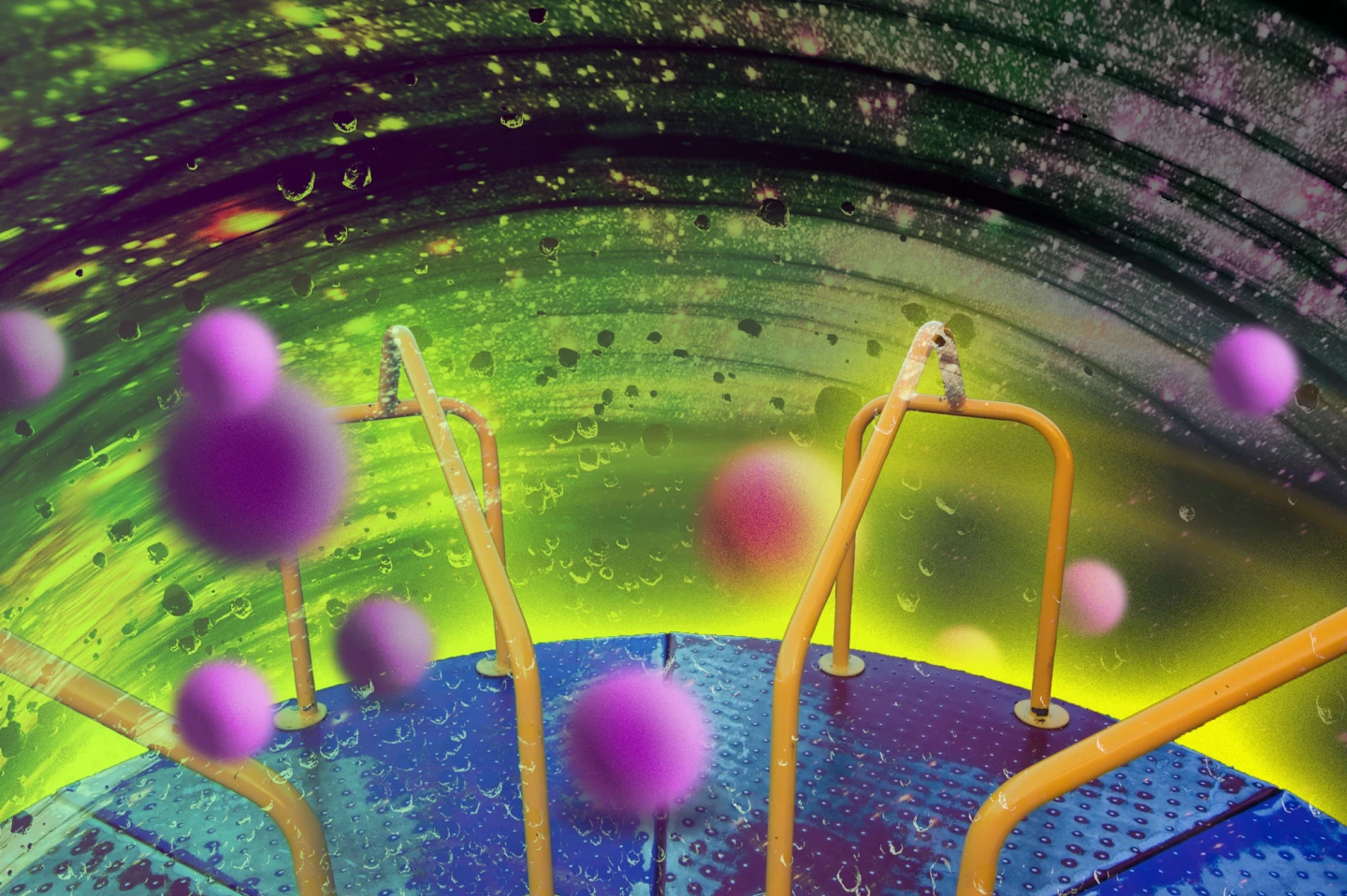
But we don’t have to just search for dark matter in our own backyard – the stuff pervades the universe, so perhaps we should be looking out into the cosmos for it.
Astronomers have suggested that a dark matter candidate called a sterile neutrino could decay into photons and normal neutrinos, giving off X-ray emissions in the process. Looking for these otherwise unexplained X-ray bursts in galaxies could be a smoking gun for dark matter.
Similarly, axions could be turned into visible photons in the extreme magnetic fields around neutron stars. Intriguingly, in early 2021 Berkeley Lab astronomers reported the detection of unusual high-energy X-ray emissions from a neutron star, which fit the bill for axions.
In another study, astronomers searched for evidence of dark matter dragging on black holes. The idea is that if a particular type of ultralight boson (which includes axions) exists, clouds of these tiny particles should gather around black holes of a certain mass, and actually slow down the rate at which they spin. Measuring the spin speed of black holes could then indicate the presence of dark matter.
Null results aren’t void
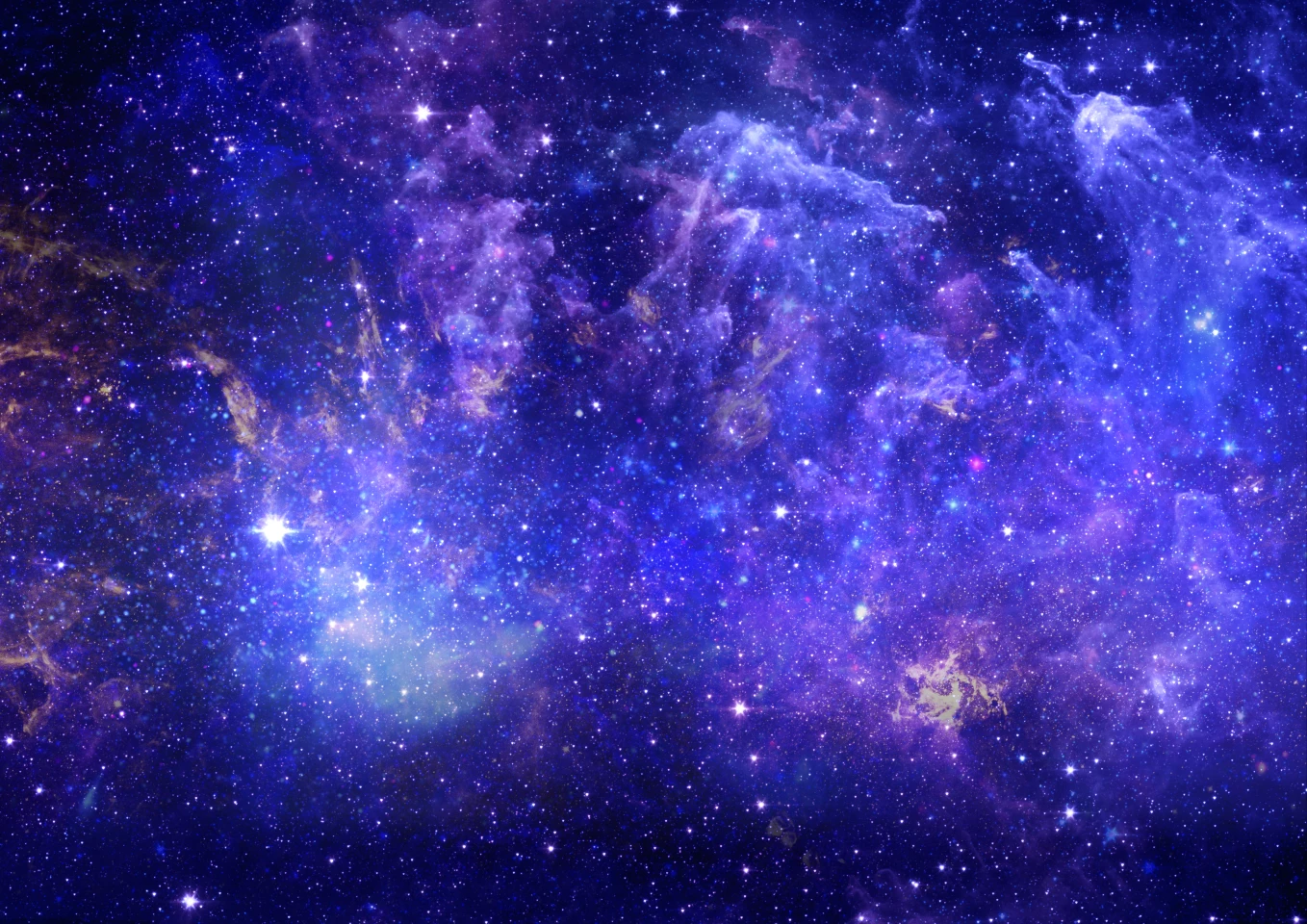
Sadly enough, all of the experiments described above have either returned null results on dark matter, or are purely theoretical for now. But not getting a signal doesn’t make an experiment a total washout – null results are important to help whittle away at that gigantic possibility space.
Each test searches for dark matter candidates within a certain mass range and with certain properties, and as we cross them off the list we’re getting ever closer to the truth. And it helps that many of the experiments are getting upgrades in the future that will make them ever-more sensitive.
In the meantime, brand new ideas are often proposed. In recent years, scientists have suggested that dark matter could take the form of superheavy gravitinos, d-star hexaquarks, or even a “dark fluid” with negative mass that permeates the universe.
Or of course, maybe it’s just a mathematical misunderstanding or if some other unseen and unknown force is creating these strange gravitational effects. Whatever it is, the hunt for dark matter is far from over, and with so many scientists searching, we might soon make one of the most important scientific discoveries of all time – or realize we’ve been led on the greatest wild goose chase ever.
Editor's note: This article was originally published on June, 2020. This revised and updated version, which covers the latest research on dark matter, was published on April 20, 2021.